- 1Department of Electronics, Information and Bioengineering, Politecnico di Milano, Milan, Italy
- 2Agenzia Regionale Emergenza Urgenza (AREU), Milan, Italy
- 3Istituto Auxologico Italiano IRCCS, Milan, Italy
Objectives: We aimed to analyze recent literature on heat effects on cardiovascular morbidity and mortality, focusing on the adopted heat definitions and their eventual impact on the results of the analysis.
Methods: The search was performed on PubMed, ScienceDirect, and Scopus databases: 54 articles, published between January 2018 and September 2022, were selected as relevant.
Results: In total, 21 different combinations of criteria were found for defining heat, 12 of which were based on air temperature, while the others combined it with other meteorological factors. By a simulation study, we showed how such complex indices could result in different values at reference conditions depending on temperature. Heat thresholds, mostly set using percentile or absolute values of the index, were applied to compare the risk of a cardiovascular health event in heat days with the respective risk in non-heat days. The larger threshold’s deviation from the mean annual temperature, as well as higher temperature thresholds within the same study location, led to stronger negative effects.
Conclusion: To better analyze trends in the characteristics of heatwaves, and their impact on cardiovascular health, an international harmonization effort to define a common standard is recommendable.
Introduction
Due to climate change, extreme weather events such as heatwaves, drought, hurricanes, and floods are becoming more frequent worldwide [1–3]. The years 2015–2021 were reported as the warmest 7 years period since 1850 [4]. Furthermore, apart from their frequency, heatwaves are also increasing in intensity and duration [5, 6], with their climatological characteristics related to rainfall and weak pressure gradient events [7, 8]. The Intergovernmental Panel on Climate Change identified the risk of death and illness from heatwaves, especially in the vulnerable population of urban areas, as one of the eight major risks related to global climate change [9].
The negative impact of high temperatures on human health has been assessed by several studies [10–12]. In particular, passive heat stress can affect the human cardiovascular (CV) system by increasing the heart rate and left ventricular contractility, and by reducing central blood volume, left ventricular filling pressures and cerebral perfusion [13]. Furthermore, people with a pre-existing CV disease have been found more vulnerable to high temperature, as it reduces the organism’s ability to thermoregulate [14]. A recent systematic review and meta-analysis provided evidence of the increased CV mortality and morbidity in heat conditions based on past data [15]. Also, other aspects were explored in literature, from the projected effects of heat on health under different climate change scenarios [16–20], to the effectiveness of heat warning systems in decreasing mortality [21].
However, despite the abundance of studies on heat effects on CV health, surprisingly, there is not a unique definition of heat or heatwave, which potentially hinders the comparison of epidemiological studies. Also, the conditions required to trigger an alert in heat warning systems vary by country, both in terms of meteorological indicators and applied thresholds [22]. As a result, this complicates the comparison of epidemiological studies dealing with the potential impact of heat on human health, due to the usage of different temperature (or thermal indices) indicators. Since the beginning of the 20th century, more than 150 thermal climate indices related to human health have been proposed in the scientific literature [23]. Several standardization attempts were performed [24–30], but they failed in the identification of a unique heat definition, even within the same geographical area.
In light of the above considerations, the objective of this study was to conduct a systematic review considering open access papers published in the last 5 years, during which time the attention towards this topic has increased worldwide. The focus of the analysis was on the effects of heat on CV health, primarily considering the applied definition of heat and, secondarily, assessing whether such definitions had an impact on the applied methodology and on the reported results.
Methods
In order to ensure transparency, reproducibility, and effectiveness, a systematic review approach [31, 32] was applied. The Preferred Reporting Items for Systematic Review and Meta-Analysis (PRISMA) guidelines [33] were followed. Three online databases of scientific literature were examined—PubMed, ScienceDirect, and Scopus—of which the first covers biomedicine and life science, while the other two provide an overview of multidisciplinary research. Databases were queried in the title, abstract, or keywords using as keywords “heat*,” “high temperature*,” “extreme weather,” paired with “cardiovascular,” “heart,” “ischemic,” “cardiac,” “infarction,” “myocardial,” “hypotension,” “hypertension.” Only journal articles written in English, published between January 2018 and September 2022, and with full text open access availability were included.
Additional filtering was performed on the basis of full-text content. First, papers without an explicit definition of heat were discarded. Then, only original studies with analytic study design (i.e., cohort study, case-control study, or case-crossover study) based on official medical records (i.e., registered deaths, hospital admissions, emergency calls) were included, excluding those based on personal perception (i.e., questionnaires). Finally, for comparison purposes, only articles that reported the results as relative risk (RR), odds ratio (OR), or incidence density ratio (IDR) with a 95% confidence interval were kept. The characteristics of the included studies can be described with the following PECO [34] statement: (P) Among humans of all ages, genders and ethnicities, what is the effect of (E) exposure to heat versus (C) non-heat conditions on (O) CV morbidity and mortality.
The analysis of the resulting articles explored the following aspects:
1) Definition of heat, considering in particular three aspects:
• Indicators: meteorological parameters and measures included in the definition.
• Methods: statistical method applied for the comparison between exposure groups.
• Comparison threshold: reference value applied to subdivide exposed and non-exposed groups.
The computed values of different heat indices, in relation to different air temperatures at the reference conditions, were also compared.
2) Main characteristics of the study design, such as the analyzed outcome, medical data source, geographic distribution, sample size, and meteorological data source.
3) Quantification of the impact on CV health, considering the statistical methods and the reported results, in relation to the applied definition of heat.
All-cause CV diseases refer to the International Classification of Diseases codes 390–459 (ICD-9-CM) or I00–I99 (ICD-10-CM). To address disease-specific analyses, the results among six groups of pathologies were grouped: myocardial infarction (ICD-10 code I21–I23), ischemic (coronary) heart disease (including chest pain; I20–I25), stroke (I60–I69), hypertensive diseases (I10–I15), heart failure (I50) and other diseases (i.e., chronic rheumatic heart disease, out-of-hospital cardiac arrest, acute aortic dissection, arrhythmias).
In the following text, the term “heat” is used as abbreviation of “heatwave,” the acronym TEMP is used for “temperature,” while the term “outcome” describes the medical result (i.e., the received diagnosis or death), and “result” refers to the RR/OR/IDR reported in the studies. If a factor results in a “negative effect on health” it means that the risk of developing a health-related negative CV event is increased. All reported plots were created with Plotly library (version 5.11.0) in Python (version 3.8.5).
Results
The adopted query resulted in the identification of 11,783 articles (3,602 in PubMed, 2,390 in ScienceDirect and 5,791 in Scopus) in the English language and published between January 2018 and September 2022. After title and/or abstract screening, 347 items were selected as possibly relevant articles, of which 232 were unique. During the phase of full text screening, 146 studies were excluded as not relevant, due to the lack of an explicit definition of heat, not focusing in the reported results on CV outcomes, or using a study design other than analytic. Lastly, considering only articles reporting results (i.e., the influence of heat on CV health) as RR, OR, or IDR with 95% confidence, 54 articles were finally selected [35–88]. Figure 1 shows the summary of this literature systematic review process.
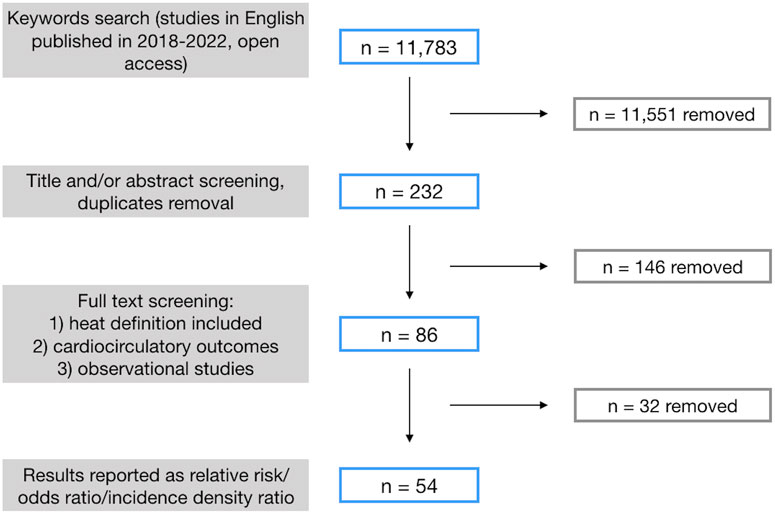
FIGURE 1. Schematic of the results of the systematic literature review process, including open access papers published between 2018 and 2022, written in the English language, and resulting from the application of the selected query (see text for details) (systematic review, international, 2018–2022).
Definition of Heat
In total, 21 different combinations of criteria to define heat were found (Table 1), with only three studies simultaneously testing and comparing more than one of them. The most frequently used meteorological measure as heat indicator was air TEMP, applied in 56 of 57 cases. In nine studies, air TEMP was included in the computation of more complex indices, such as “Apparent Temperature” [42, 53, 60, 71, 76, 83], “Perceived Temperature” [39], “Pseudo-equivalent daily temperature” [88] or “Discomfort Score” [56], by combining it with relative humidity, water vapor pressure, dew point TEMP, wind speed and/or heat radiation. The relevant calculation formulas are summarized in Table 2, except for “Perceived Temperature” [39] as it was not provided in the relevant study. Only one study [74] used air masses (excluding air TEMP) to define heat days.
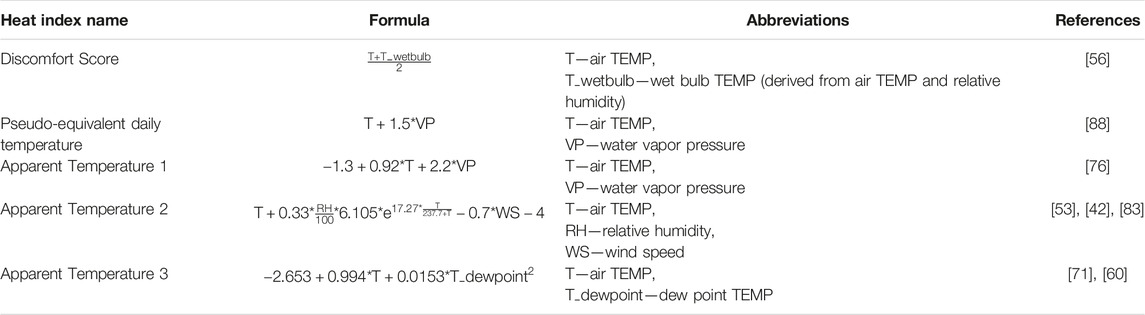
TABLE 2. Calculation formulas for complex heat indices (systematic review, international, 2018–2022).
In the majority of cases (n = 46), heat days were defined using thresholds on percentiles of TEMP distribution over a certain period, with TEMP defined by daily mean, minimum or maximum values. The three most frequent thresholds were 99th (n = 17), 95th (n = 13) and 97.5th (n = 11) percentiles. In eight cases, the thresholds were set on absolute TEMP values, in the range 20°C–35°C. In several studies, the threshold criterion was coupled with a duration requirement (e.g., TEMP above the threshold for at least 2–5 days). When the study period was limited to the warmer months only, the considered TEMP distribution was either annual or periodic. The lowest value of heat threshold reported in literature was a mean daily temperature of 20°C [39], set in Austria, while the highest was a mean daily temperature of 43°C, set in Kuwait [73].
As regards the threshold-based identification of the non-exposed groups for comparison, this was selected based on:
• the minimum mortality TEMP (MMT) or its equivalent (minimum hospital admission TEMP, minimum ambulance calls TEMP etc.), derived from the relevant minimum mathematical function applied to the temporal series.
• another percentile (i.e., the 50th, 75th, 90th of the TEMP distribution or the 1st percentile of heat index values).
• defining non-heatwave days (i.e., all days not classified as heat).
• mean TEMP or arbitrary absolute value.
Moreover, in one study, instead of a threshold for direct comparison, the authors analyzed the effects of a 1°C increase in TEMP above the selected threshold [61].
It is worth noting that in four studies the heat definitions were used at a national level for heat warning systems (Table 3).
For a deeper analysis and visual comparison of the different heat indexes summarized in Table 2, in Figure 2 they were plotted as a function of air TEMP, with the other parameters arbitrarily set to standard conditions (relative humidity = 50%, wind speed = 1 m/s, water vapor pressure = 2.34 kPa, dry bulb TEMP equal to air TEMP and dew point TEMP calculated according to Lawrence [90]).
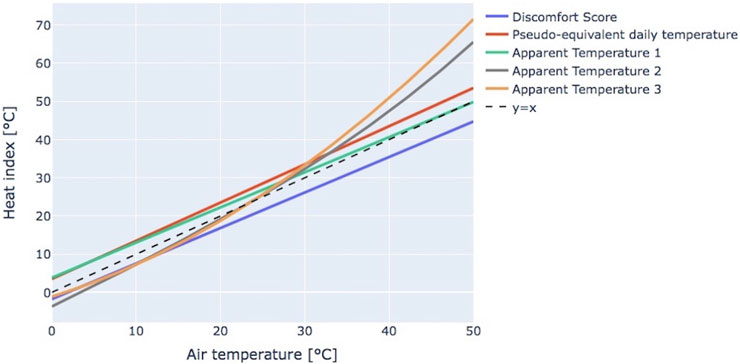
FIGURE 2. Heat indexes as a function of temperature as defined in Table 2, computed assuming relative humidity = 50%, wind speed = 1 m/s, water vapor pressure = 2.34 kPa and dew point temperature calculated according to Lawrence [90] (systematic review, international, 2018–2022).
From this graph, it can be noted that, in colder environments (i.e., 0°C–20°C), Pseudo-equivalent daily temperature and Apparent Temperature 1, based on water vapor pressure, reach significantly higher values compared to the other indexes, with a maximum difference up to 7°C. When the air TEMP is closer to 30°C, which is considered in many studies as the threshold for heat days, there are no big differences among the indexes, except the Discomfort Score, characterized by lower values. Above a value of air TEMP equal to 30.4°C, the differences among indexes increase progressively, with Apparent Temperature 2 and 3 rising to higher values due to their exponential/polynomial formulas.
Study Characteristics
Among the selected articles, 27 (50%) were targeting the analysis of mortality, 25 (46.3%) of morbidity and 2 (3.7%) of both these outcomes (Supplementary Figure S1).
Among the studies including morbidity as a target, the majority (n = 18) referred to hospital admissions (including three on emergency hospital admissions), while the rest considered emergency calls (n = 4), emergency department visits (n = 3), chest pain centre visits (n = 1), or general practitioner consultations (n = 1).
In 21 (38.9%) studies, only all-cause CV diagnoses were considered, while in the remaining 33 (61.1%) studies the results were stratified by specific CV disease. Additionally, regardless of the target outcome, in a few studies the impact of heat was also studied on specific subgroups, stratified by age (9 studies; 16.7%), by age adjusted for exposure to noise or chronic conditions (2 studies; 3.7%), or by the presence of a chronic condition alone (1 study; 1.9%).
The geographical origin of the selected studies spanned areas from all six continents, with 25 (46.3%) articles from Asia, 15 (27.8%) from Europe, 8 (14.8%) from North America, 3 (5.6%) from Oceania, 2 (3.7%) from South America and 1 (1.9%) from Africa. The country covered with the highest number of studies was China, using either data from single cities [42, 52, 53, 65, 75, 83, 87], individual provinces [46, 48, 68], or multiple cities spread throughout the country [37, 47, 86].
The sample size of the target study group was explicitly reported in 40 (74.1%) articles; alternatively, it was inferred by multiplying the average daily incidence by the study period when both characteristics were specified in the article (n = 10; 18.5%); in 4 (7.4%) studies it was not possible to infer any information about sample size. The median sample size was slightly above 40,000 subjects, with a minimum of 939 individuals in a study about congestive heart failure hospital admissions and mortality in Boston, New York and Philadelphia [85], and a maximum of 1,154,896 in an analysis of all-cause CV morbidity in South Africa [84]. Sample size distribution in the different geographical areas is reported in Supplementary Figure S2.
The median observation period was 10 years, with a minimum of 2 years for Israel [56], Slovenia [88] and a Chinese study [83], and a maximum of 28 years for Germany [57]. Several studies (n = 22; 40.7%) analyzed the influence of heat only in an arbitrarily defined warmer period of the year, beginning within March–June and ending within June–October for the Northern Hemisphere, while spanning from September-November to March for the Southern Hemisphere. When narrowing the study period, the most frequent interval was May-September (n = 9; 16.7%).
Regarding the use of meteorological data as explicative attributes in the analysis, dew point/air TEMP, relative humidity, wind speed, atmospheric/barometric pressure, precipitation, sunshine duration, solar radiation and air pollution were utilized. When the mean values for each factor were reported (n = 36; 66.7%), they were calculated by averaging all records from all measurement stations in the study area. In 9 (16.7%) studies, interpolated meteorological maps (e.g., maps at ZIP code level [82], district level [84] or at 2 km spatial resolution [36]) were alternatively used.
Among the 13 (24.1%) studies for which the events’ location or patients’ home address were available, only two [48, 56] associated them with the meteorological conditions at the event time, while Jiang et al. [86] linked the TEMP with that at the hospital location, as the majority of patients did not report their complete address.
Quantification of Heat Impact on Cardiovascular Health
To assess the impact of heat on CV health, the majority of articles (n = 39; 72.2%) applied the distributed lag non-linear model, which describes the response to exposure accounting for a delayed effect [91]. The number of tested lags varies up to 30 days, with the most frequent choices being 21 (n = 15; 27.8%), 3 (n = 9; 16.7%), and 14 (n = 5; 9.3%).
Six studies considered the duration of the temperature increase as a criterion to classify a heatwave, but only in two studies was a stronger negative effect reported. Other applied techniques included the generalized linear models (n = 13; 24.1%), or a simplified approach based on the comparison between incidence rates during heat and non-heat days (n = 2; 3.7%). In several studies, mostly in those using only air TEMP to define heat, the statistical models were further adjusted by meteorological factors (n = 9; 16.7%), by air pollution (n = 4; 7.4%) or by both (n = 22; 40.7%).
In 14 studies (25.9%) a case-crossover design, as proposed by Maclure [92], was applied. By using the same subjects as both cases and control at different points in time, this design allows to control for time-invariant characteristics, such as gender or medical history.
In the majority of studies, the results were reported as RR (n = 44; 81.5%), and in the remaining studies as OR (n = 7; 13%) or IDR (n = 3; 5.6%). The values of these three indicators are approximately equal when the initial risk (i.e., prevalence of the disease in the population under study in non-heat conditions) is relatively small [35, 93, 94], and are therefore comparable under this assumption.
Most of the results (n = 37, 68.5%) showed a negative influence of heat for all-causes CV health (i.e., an increase in risk in relation to the comparison threshold as indicated in Table 1) in the range from 1.02 in Spain (95% CI: [1.00–1.04]) [70] and New York State (95% CI: [1.01–1.04]) [58] to 1.47 (95% CI: [1.43–1.51]) in Jiangsu Province, China [48], with two outliers equal to 4.61 (95% CI: [3.67–5.78]) [69] and 3.09 (95% CI: [1.72–5.55]) [73]. However, the studies differed in the applied heat definition (Table 1). All studies that reported a negative effect of heat on CV health found this effect stronger if associated with a shorter lag, while this relation seemed to weaken over time. In disease-specific analysis, the most consistent and significant results were reported for stroke, varying from 1.20 (95% CI: [1.02–1.40]) [79] to 1.62 (95% CI: [1.39–1.88]) [46], with the most frequently associated heat definition based on the combinations of air TEMP, percentile, and MMT equivalent. The strongest disease-specific result was found for myocardial infarction (5.22, 95% CI: [2.14–12.73]), with heat defined using Apparent Temperature 2, percentile and MMT equivalent [53].
However, in 11 studies (20.4%) no statistically significant results were found, while in 6 (11.1%) a statistically significant decrease in the risk of developing a CV acute event during heat was surprisingly reported. Of these six, one study analyzed all CV diseases together [83], while the others referred to specific diseases, i.e., myocardial infarction [79], hypertensive diseases [76, 79], heart failure [45, 63, 79], and acute aortic dissection [75].
A complete summary of these results is presented in Supplementary Figure S3 for all-cause CV outcomes and in Supplementary Table S1 for specific CV diseases.
Finally, to identify a possible correlation between the applied definition of heat and the reported results relevant to the CV risk, an additional analysis was performed considering the percentage deviation of the reported heat threshold from the mean annual temperature, calculated as:
This computation was feasible in 13 (24.1%) studies in which the absolute values of both parameters were explicitly specified, and relevant results are presented in Figure 3. Excluding three outliers (i.e., all-cause CV mortality in Kuwait [70], out-of-hospital cardiac arrest events in Israel [56], and acute aortic dissection morbidity in China [75]), a slight trend of higher risk with increasing deviation of the heat threshold from the mean annual temperature could be observed, yet not with robust statistical evidence, with the coefficient estimated by ordinary least squares linear regression equal to 0.002 (R2 = 0.19).
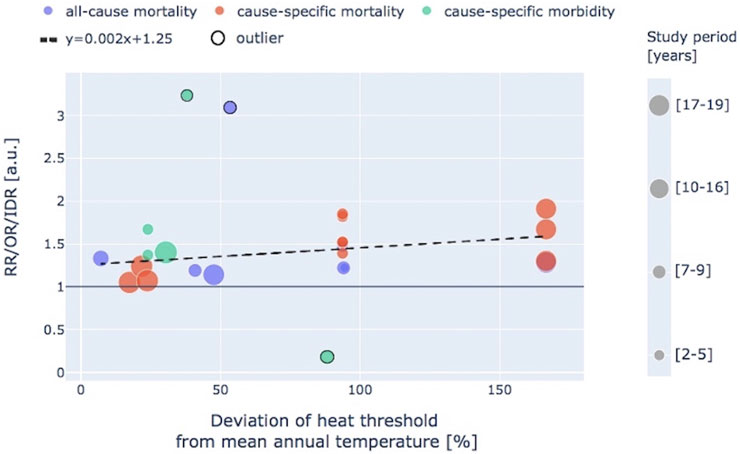
FIGURE 3. Relationship between the percentage deviation of the considered heat threshold from the mean annual temperature and associated cardiovascular risk, subdivided as all-cause and cause-specific mortality or morbidity. RR, relative risk; OR, odds ratio; IDR, incidence density ratio. Dot size is proportional to the duration of the observation period expressed as number of years (systematic review, international, 2018–2022).
Discussion
In this systematic review, studies focusing on the impact of heat on CV morbidity and/or mortality published in the last 5 years were selected, in order to assess the latest progresses made by the scientific community in this field. The identified studies were conducted across all six continents, with a leading role of Asia (both in terms of number of publications and of sample size), followed by Europe and North America. Source data spanned from 1987 to 2021.
Despite having the same goal, the examined study settings were extremely heterogeneous. Such heterogeneity could be noticed both in the definition of heat in the field of CV health, but also in other aspects, such as the medical and meteorological data used, the sample size, the observation periods, and the applied methodology for analysis. In addition, biases due to measurement error [95] or spatial autocorrelation [96] could affect data generation and processing.
Applied Definitions of Heat
As early as the beginning of the 21st century, Robinson [25] pointed out that, due to the lack of an unequivocal heat definition, it was difficult to assess if heatwaves varied in intensity or prevalence, which further hindered research into climate change. The majority of heat indices developed since the beginning of the 20th century have been collected and organically presented in a theoretical framework [23]. However, to the best of our knowledge, a comparison of their viability in health-related studies is still missing. Moreover, only a small fraction of those indices uses exclusively meteorological factors, such as air TEMP, relative humidity and solar radiation [97] or air TEMP, dewpoint TEMP, relative humidity, cloud cover, and wind speed [98], whereas many other indices (e.g., [99–103]) are also based on human thermal comfort factors (i.e., human metabolic rate), a type of data that is labor-intensive in terms of collection and processing, thus hindering their application in heat-health related research. In the examined recent literature, only one review focusing on heat definitions and addressing their heterogeneity was found [104]. It included 60 studies published in 2001–2015 covering Asia, Australia, Europe, and North America, considering the impact of heat on all-cause CV and respiratory mortality.
In line with this established knowledge, twenty-one unique combinations of indicators, methods, and comparison thresholds, with the purpose of defining heat days and to distinguish them from non-heat days, were identified in the studies selected for this review. Differently from de Freitas and Grigorieva [23], the majority of these studies used air TEMP alone, averaging the values obtained from measurement stations without applying spatial interpolation. Other meteorological data (i.e., relative humidity, water vapor pressure, dew point TEMP, wind speed and/or heat radiation) were eventually used only for a following adjustment of the statistical models.
Several different approaches could be identified:
- even when a combined indicator was applied, it is worth noting that three different versions of “Apparent Temperature” were found, as a result of the application of simplified versions, depending on data availability, to the same original index [23].
- only the term “Perceived Temperature” can be referred directly to a specific and standardized heat index.
The choice of the index to be considered for the analysis is strongly relevant in terms of comparing results obtained in different studies. In fact, as shown in Figure 2, the values of the different indexes as a function of TEMP cannot be assumed to be the same, except for a narrow range around 30°C.
Two main methods for setting the heat threshold were identified, namely, using a percentile of the indicator’s distribution or its absolute value. However, in this latter case, the reported threshold values varied in a range of 15°C, making it impossible to formulate a universal threshold with this strategy. In addition, four national definitions implemented in heat warning systems were identified, one of which applied to a territory different from the country in which the criterion was defined. Our findings, showing strong heterogeneity in heat definitions applied to research studied published from 2018 to 2022, indicated that despite the increased international interest in climate change effects on health, there was no important progress in the harmonization and standardization process towards a singular heat definition to be applied in the context of CV health. A similar finding was already present in a former systematic review including 60 studies published in the period 2001–2015 [104], thus further highlighting the lack of action of the scientific community towards this problem.
It is important to assess if there is an overall trend, transversal to different settings, in the relationship between the selected definition of heat and the related results in terms of impact on CV health. In this perspective, Xu et al. [104] noted that a modification of the threshold value led to a more important change in mortality than the modification of heatwave duration, which is consistent with our findings. In studies where multiple thresholds were tested, a higher risk was usually reported for higher thresholds. This trend is in agreement with Xu et al. [105], who focused on heat definitions and five health events (i.e., ambulance service uses, all-cause and cause-specific emergency department attendances, all-cause and cause-specific hospitalizations) in Brisbane, Australia. In that study, multiple heat definitions were tested, combining three durations (2–4 days) and 10 percentiles (90th–99th) applied on mean and maximum air TEMP. The authors concluded that, for three of these five health events, a threshold selection with the 97th percentile resulted in a significant association with heat (regardless of duration and TEMP indicator), while for the other two a significant correlation was found with a threshold set to 98th or 99th percentile (depending on TEMP indicator). Among the studies included in this review, it is worth noting that two outliers with very elevated health risk in all-cause CV events were identified for Seville and Kuwait. The former compared the 99th percentile of a heat index distribution, accounting for the TEMP in time windows of up to 30 days, to its 1st percentile. The latter compared the 99th percentile of daily mean air TEMP distribution to the MMT. In both geographical areas, a very high percentile threshold was considered even though both of these territories are characterized by particularly hot summers, thus focusing on extreme events only, in a set-up that could partly explain the outlier results.
Impact of Heat on Cardiovascular Health
As a secondary target, this review also focused on the results of the analysis conducted in the included studies, with an aim of assessing the impact of heat on CV health, thus addressing the applicability and robustness of heat definitions towards this specific purpose.
On this topic, Xu et al. [104] concluded, pooling the results of their review, that an increased mortality could be found in the heat condition. Moreover, in the United States, the relationship between temperature and all-cause mortality was found to be non-stationary from a spatial point of view [106]. In previous research on the effect of heat on mortality and morbidity, both individual (e.g., age, gender, medical history, race, education level, occupation) and community-level (e.g., housing quality, medical care, air pollution) characteristics were identified as effect modifiers [107–112]. In this regard, the case-crossover method should present an advantage over traditional cohort or case-study designs, as it overcomes the between-person confounding factors. In the studies here considered, the vast majority of results reported a negative impact of heat on all-cause CV health, yet they used different parameters (mainly RR, but also OR and IDR) computed from different data sources (hospital admissions, emergency calls, emergency visits). In general, no specific information on the patient’s home address could be matched with the meteorological conditions at the time of the event.
In CV disease-specific analysis of both morbidity and mortality, an increased risk for stroke and ischemic (coronary) heart disease was evidenced in all the examined studies. In the case of the former, similar results have been previously reported, being particularly strong for ischemic stroke [113, 114], with possible explanations including dehydration, increased blood viscosity, hemoconcentration, and elevated cholesterol levels during heat [113]. In addition, indications that a sudden and large increase in TEMP (i.e., ∆TEMP ≥ 5°C) increases the risk of ischemic stroke events, rather than the absolute TEMP values, were given [115].
The increased risk of coronary artery disease could be explained with endothelial dysfunction expressed as a reduction of flow-mediated vasodilation associated with increased temperature [116]. When patients are exposed to a hot environment, the CV system increases the cardiac output to augment blood flow to the skin for cooling [117], thus increasing the heart oxygen consumption and potentially generating a precipitation of events towards myocardial infarction. An increased risk for this pathology was reported in 11/12 analyzed studies. Similarly, a previous study demonstrated that higher environmental temperatures were associated with an increased risk in myocardial infarction [118], with an influential effect during the first 6 h after exposure.
The increased risk found in 5/7 studies on hypertension-related outcomes may seem contradictory as a response to heat, but it was explained with a possible interaction between heat and anti-hypertensive medications [79].
When focusing on heart failure morbidity, a lower risk was apparently associated with heat. However, one of these studies also analyzed heart failure mortality and reported a higher RR. This finding is in agreement with other reviews on the effects of heat on CV diseases, where a predominance of negative effects was reported for mortality, in contrast with an impact on morbidity that was not always consistent [15, 119–121].
Among other causes, risk of aortic dissection was found to be reduced with heat, while risks of out-of-hospital cardiac arrest, chronic rheumatic heart disease, and arrythmias were found to be increased.
Study Limitations and Conclusion
Our study has some limitations. Despite the selection of studies being conducted with the most uniform approach possible with regards to the methodology, the heterogeneity of resulting articles made any advanced meta-analysis unfeasible. The dissimilarities did not only concern the main target of this work, which was the definition of heat, but also affected other factors as well, such as the health outcomes or the characteristics of the studied population. The attempt to generalize the results, neglecting details such as the considered effect modifiers, may have led to some particular trends being missed. Moreover, studying heat and heatwaves has consistent and complex climatological implications that were not considered in detail in this review, as the focus was limited to the effects of heat on cardiovascular health. Lastly, an inherent limitation of our study is that it considered only open access publications over an arbitrarily selected short time frame of 5 years. However, this choice was made to focus on more recent studies to capture the state-of-the-art relevant to the topic, as a previous systematic review by Xu et al. (2016) covered previously published studies. As the literature search and data extraction were performed by a single person, this constitutes a possible methodological limitation of our approach.
Despite the abundance of recent research in this field, there is no single definition of heat, together with a possible confusion introduced by the terminology and related different formulas for the utilized heat indices. An international effort should be conducted in order to harmonize a common standard in representing and evaluating such data including the definition of heatwave. This would allow better comparison among geographical areas and analysis of trends in heatwave intensity, duration and prevalence, as well as the human heat adaptation capabilities. Such analyses could be incorporated into public health programs with a two-fold strategy: short-term intervention, by developing automated systems capable of analyzing and predicting, based on the current environmental variables on a given territory, the possible immediate impact of heatwaves, to guide emergency services in a better organization and deployment of available resources, as well as activating heat alert systems; and mid-long term intervention, by highlighting those features for a certain territory that could influence the citizen’s resilience, and planning urbanistic interventions accordingly.
Author Contributions
Study conception and design: EC, JN, and LG; data collection: JN; analysis and interpretation of results: JN, LG, AP, GS, and EC; draft manuscript preparation: EC, JN, and LG. All authors contributed to the article and approved the submitted version.
Funding
JN was supported by a Ph.D. Fellowship from “PON Research and Innovation 2014–2020 Action IV.5”. EC was supported by the Italian project “Anthem”. This work was funded by the National Plan for NRRP Complementary Investments (PNC, established with the decree-law 6 May 2021, n. 59, converted by law n. 101 of 2021) in the call for the funding of research initiatives for technologies and innovative trajectories in the health and care sectors (Directorial Decree n. 931 of 06-06-2022)—project n. PNC0000003—AdvaNced Technologies for Human-centrEd Medicine (project acronym: ANTHEM).
Author Disclaimer
This work reflects only the authors’ views and opinions, neither the Ministry for University and Research nor the European Commission can be considered responsible for them.
Conflict of Interest
The authors declare that they do not have any conflicts of interest.
Supplementary Material
The Supplementary Material for this article can be found online at: https://www.ssph-journal.org/articles/10.3389/phrs.2023.1606266/full#supplementary-material
Supplementary Figure S1 | Summary of articles by target outcome (systematic review, international, 2018–2022).
Supplementary Figure S2 | Summary of selected articles divided by continent of interest for the analysis, stratified by sample size of the examined study group; in 10 (18.5%) studies, the sample size was inferred considering the average incidence and the study period (systematic review, international, 2018–2022).
Supplementary Figure S3 | Summary of the influence of heat on all-cause cardiovascular outcomes. The notation e in the last column means that the sample size was inferred as not directly specified in the relevant study. The letters a–l refer to the combination of criteria used to define heat: a – air TEMP + percentile + MMT or equivalent, b – air TEMP + percentile and duration + non-heatwave, c – air TEMP+relative humidity+wind speed + percentile + MMT or equivalent, d – air TEMP + percentile + non-heatwave, f – air temperature + dew point temperature + percentile + MMT or equivalent, g – air TEMP + percentile + another percentile, h – air TEMP + absolute value and duration + non-heatwave, i – air TEMP + other + another percentile, j – air TEMP+relative humidity+wind speed + percentile + another percentile, k – air TEMP + absolute value + MMT or equivalent, l – air TEMP+water vapor pressure + absolute value and duration + non-heatwave (systematic review, international, 2018–2022).
Supplementary Table S1 | Summary of the influence of heat on cause-specific cardiovascular outcomes. The notation e in the “Sample size” column means that the sample size was inferred as not directly specified in the relevant study (systematic review, international, 2018–2022).
References
1. Maxwell, SL, Butt, N, Maron, M, McAlpine, CA, Chapman, S, Ullmann, A, et al. Conservation Implications of Ecological Responses to Extreme Weather and Climate Events. Divers Distributions (2019) 25(4):613–25. doi:10.1111/ddi.12878
2. National Academies of Sciences. Engineering, and Medicine. In: Attribution of Extreme Weather Events in the Context of Climate Change. Washington, D.C: National Academies Press (2016).
3. Ummenhofer, CC, and Meehl, GA. Extreme Weather and Climate Events With Ecological Relevance: A Review. Phil Trans R Soc B: Biol Sci (2017) 372(1723):20160135. doi:10.1098/rstb.2016.0135
4. World Meteorological Organization. State of the Global Climate 2021. Switzerland: World Meteorological Association (2022).
5. Perkins-Kirkpatrick, SE, and Lewis, SC. Increasing Trends in Regional Heatwaves. Nat Commun (2020) 11(1):3357. doi:10.1038/s41467-020-16970-7
6. Steffen, W, Hughes, L, and Perkins, S. Heatwaves: Hotter, Longer, More Often. Climate Council of Australia (2014).
7. Spensberger, C, Madonna, E, Boettcher, M, Grams, CM, Papritz, L, Quinting, JF, et al. Dynamics of Concurrent and Sequential Central European and Scandinavian Heatwaves. Q J R Meteorol Soc (2020) 146(732):2998–3013. doi:10.1002/qj.3822
8. Hunt, BG. A Climatology of Heat Waves From a Multimillennial Simulation. J Clim (2007) 20(15):3802–21. doi:10.1175/jcli4224.1
9. IPCC. In: H-O Pörtner, DC Roberts, M Tignor, ES Poloczanska, K Mintenbeck, A Alegríaet al. editors Climate Change 2022: Impacts, Adaptation and Vulnerability. Contribution of Working Group II to the Sixth Assessment Report of the Intergovernmental Panel on Climate Change. Cambridge: Cambridge University Press. (2022). p. 3056.
10. Liu, J, Varghese, BM, Hansen, A, Xiang, J, Zhang, Y, Dear, K, et al. Is There an Association Between Hot Weather and Poor Mental Health Outcomes? A Systematic Review and Meta-Analysis. Environ Int (2021) 153:106533. doi:10.1016/j.envint.2021.106533
11. Ballester, J, Quijal-Zamorano, M, Méndez Turrubiates, RF, Pegenaute, F, Herrmann, FR, Robine, JM, et al. Heat-Related Mortality in Europe During the Summer of 2022. Nat Med (2023) 29:1857–66. doi:10.1038/s41591-023-02419-z
12. Schneider, A, and Breitner, S. Temperature Effects on Health-Current Findings and Future Implications. EbioMedicine (2016) 6:29–30. doi:10.1016/j.ebiom.2016.04.003
13. Crandall, CG, and Gonzalez-Alonso, J. Cardiovascular Function in the Heat-Stressed Human. Acta Physiol (2010) 199(4):407–23. doi:10.1111/j.1748-1716.2010.02119.x
14. Zhang, X, Noda, S, Himeno, R, and Liu, H. Cardiovascular Disease-Induced Thermal Responses During Passive Heat Stress: An Integrated Computational Study. Int J Numer Methods Biomed Eng (2016) 32(11):e02768. doi:10.1002/cnm.2768
15. Liu, J, Varghese, BM, Hansen, A, Zhang, Y, Driscoll, T, Morgan, G, et al. Heat Exposure and Cardiovascular Health Outcomes: A Systematic Review and Meta-Analysis. Lancet Planet Health (2022) 6(6):e484–e495. doi:10.1016/S2542-5196(22)00117-6
16. Dong, W, Liu, Z, Liao, H, Tang, Q, and Li, XE. New Climate and Socio-Economic Scenarios for Assessing Global Human Health Challenges Due to Heat Risk. Climatic Change (2015) 130(4):505–18. doi:10.1007/s10584-015-1372-8
17. Gu, S, Zhang, L, Sun, S, Wang, X, Lu, B, Han, H, et al. Projections of Temperature-Related Cause-Specific Mortality Under Climate Change Scenarios in a Coastal City of China. Environ Int (2020) 143:105889. doi:10.1016/j.envint.2020.105889
18. Hajat, S, Vardoulakis, S, Heaviside, C, and Eggen, B. Climate Change Effects on Human Health: Projections of Temperature-Related Mortality for the UK During the 2020s, 2050s and 2080s. J Epidemiol Community Health (2014) 68(7):641–8. doi:10.1136/jech-2013-202449
19. Onozuka, D, Gasparrini, A, Sera, F, Hashizume, M, and Honda, Y. Future Projections of Temperature-Related Excess Out-of-Hospital Cardiac Arrest Under Climate Change Scenarios in Japan. Sci Total Environ (2019) 682:333–9. doi:10.1016/j.scitotenv.2019.05.196
20. Rodrigues, M, Santana, P, and Rocha, A. Statistical Modelling of Temperature-Attributable Deaths in Portuguese Metropolitan Areas Under Climate Change: Who Is at Risk? Atmosphere (2020) 11(2):159. doi:10.3390/atmos11020159
21. Toloo, G, FitzGerald, G, Aitken, P, Verrall, K, and Tong, S. Evaluating the Effectiveness of Heat Warning Systems: Systematic Review of Epidemiological Evidence. Int J Public Health (2013) 58(5):667–81. doi:10.1007/s00038-013-0465-2
22. Casanueva, A, Burgstall, A, Kotlarski, S, Messeri, A, Morabito, M, Flouris, AD, et al. Overview of Existing Heat-Health Warning Systems in Europe. Int J Environ Res Public Health (2019) 16(15):2657. doi:10.3390/ijerph16152657
23. de Freitas, CR, and Grigorieva, EA. A Comprehensive Catalogue and Classification of Human Thermal Climate Indices. Int J biometeorology (2015) 59(1):109–20. doi:10.1007/s00484-014-0819-3
24. Perkins, SE, and Alexander, LV. On the Measurement of Heat Waves. J Clim (2013) 26(13):4500–17. doi:10.1175/jcli-d-12-00383.1
25. Robinson, PJ. On the Definition of a Heat Wave. J Appl Meteorology Climatology (2001) 40(4):762–75. doi:10.1175/1520-0450(2001)040<0762:otdoah>2.0.co;2
26. Smith, TT, Zaitchik, BF, and Gohlke, JM. Heat Waves in the United States: Definitions, Patterns and Trends. Climatic change (2013) 118(3):811–25. doi:10.1007/s10584-012-0659-2
27. You, Q, Jiang, Z, Kong, L, Wu, Z, Bao, Y, Kang, S, et al. A Comparison of Heat Wave Climatologies and Trends in China Based on Multiple Definitions. Clim Dyn (2017) 48(11):3975–89. doi:10.1007/s00382-016-3315-0
28. Chen, K, Bi, J, Chen, J, Chen, X, Huang, L, and Zhou, L. Influence of Heat Wave Definitions to the Added Effect of Heat Waves on Daily Mortality in Nanjing, China. Sci Total Environ (2015) 506:18–25. doi:10.1016/j.scitotenv.2014.10.092
29. Kang, C, Park, C, Lee, W, Pehlivan, N, Choi, M, Jang, J, et al. Heatwave-Related Mortality Risk and the Risk-Based Definition of Heat Wave in South Korea: A Nationwide Time-Series Study for 2011–2017. Int J Environ Res Public Health (2020) 17(16):5720. doi:10.3390/ijerph17165720
30. Tong, S, FitzGerald, G, Wang, XY, Aitken, P, Tippett, V, Chen, D, et al. Exploration of the Health Risk-Based Definition for Heatwave: A Multi-City Study. Environ Res (2015) 142:696–702. doi:10.1016/j.envres.2015.09.009
31. Aromataris, E, and Pearson, A. The Systematic Review: An Overview. AJN Am J Nurs (2014) 114(3):53–8. doi:10.1097/01.NAJ.0000444496.24228.2c
32. Wright, RW, Brand, RA, Dunn, W, and Spindler, KP. How to Write a Systematic Review. Clin Orthopaedics Relat Res (2007) 455:23–9. doi:10.1097/BLO.0b013e31802c9098
33. Page, MJ, McKenzie, JE, Bossuyt, PM, Boutron, I, Hoffmann, TC, Mulrow, CD, et al. The PRISMA 2020 Statement: An Updated Guideline for Reporting Systematic Reviews. Int J Surg (2021) 88:105906. doi:10.1016/j.ijsu.2021.105906
34. Morgan, RL, Whaley, P, Thayer, KA, and Schünemann, HJ. Identifying the PECO: A Framework for Formulating Good Questions to Explore the Association of Environmental and Other Exposures With Health Outcomes. Environ Int (2018) 121(1):1027–31. doi:10.1016/j.envint.2018.07.015
35. Alsaiqali, M, De Troeyer, K, Casas, L, Hamdi, R, Faes, C, and Van Pottelbergh, G. The Effects of Heatwaves on Human Morbidity in Primary Care Settings: A Case-Crossover Study. Int J Environ Res Public Health (2022) 19(2):832. doi:10.3390/ijerph19020832
36. Saucy, A, Ragettli, MS, Vienneau, D, de Hoogh, K, Tangermann, L, Schäffer, B, et al. The Role of Extreme Temperature in Cause-Specific Acute Cardiovascular Mortality in Switzerland: A Case-Crossover Study. Sci Total Environ (2021) 790:147958. doi:10.1016/j.scitotenv.2021.147958
37. Yin, P, Chen, R, Wang, L, Liu, C, Niu, Y, Wang, W, et al. The Added Effects of Heatwaves on Cause-Specific Mortality: A Nationwide Analysis in 272 Chinese Cities. Environ Int (2018) 121:898–905. doi:10.1016/j.envint.2018.10.016
38. Li, Y, Akkus, C, Yu, X, Joyner, A, Kmet, J, Sweat, D, et al. Heatwave Events and Mortality Outcomes in Memphis, Tennessee: Testing Effect Modification by Socioeconomic Status and Urbanicity. Int J Environ Res Public Health (2019) 16(22):4568. doi:10.3390/ijerph16224568
39. Kienbacher, CL, Kaltenberger, R, Schreiber, W, Tscherny, K, Fuhrmann, V, Roth, D, et al. Extreme Weather Conditions as a Gender-Specific Risk Factor for Acute Myocardial Infarction. Am J Emerg Med (2021) 43:50–3. doi:10.1016/j.ajem.2021.01.045
40. Huang, C, Cheng, J, Phung, D, Tawatsupa, B, Hu, W, and Xu, Z. Mortality Burden Attributable to Heatwaves in Thailand: A Systematic Assessment Incorporating Evidence-Based Lag Structure. Environ Int (2018) 121:41–50. doi:10.1016/j.envint.2018.08.058
41. Campbell, SL, Remenyi, TA, Williamson, GJ, White, CJ, and Johnston, FH. The Value of Local Heatwave Impact Assessment: A Case-Crossover Analysis of Hospital Emergency Department Presentations in Tasmania, Australia. Int J Environ Res Public Health (2019) 16(19):3715. doi:10.3390/ijerph16193715
42. Liu, X, Kong, D, Fu, J, Zhang, Y, Liu, Y, Zhao, Y, et al. Association Between Extreme Temperature and Acute Myocardial Infarction Hospital Admissions in Beijing, China: 2013–2016. PLoS One (2018) 13(10):e0204706. doi:10.1371/journal.pone.0204706
43. Dang, TAT, Wraith, D, Bambrick, H, Dung, N, Truc, TT, Tong, S, et al. Short-Term Effects of Temperature on Hospital Admissions for Acute Myocardial Infarction: A Comparison Between Two Neighboring Climate Zones in Vietnam. Environ Res (2019) 175:167–77. doi:10.1016/j.envres.2019.04.023
44. Bai, L, Li, Q, Wang, J, Lavigne, E, Gasparrini, A, Copes, R, et al. Increased Coronary Heart Disease and Stroke Hospitalisations From Ambient Temperatures in Ontario. Heart (2018) 104(8):673–9. doi:10.1136/heartjnl-2017-311821
45. Parry, M, Green, D, Zhang, Y, and Hayen, A. Does Particulate Matter Modify the Short-Term Association Between Heat Waves and Hospital Admissions for Cardiovascular Diseases in Greater Sydney, Australia? Int J Environ Res Public Health (2019) 16(18):3270. doi:10.3390/ijerph16183270
46. Ma, Y, Zhou, L, and Chen, K. Burden of Cause-Specific Mortality Attributable to Heat and Cold: A Multicity Time-Series Study in Jiangsu Province, China. Environ Int (2020) 144:105994. doi:10.1016/j.envint.2020.105994
47. Chen, R, Yin, P, Wang, L, Liu, C, Niu, Y, Wang, W, et al. Association Between Ambient Temperature and Mortality Risk and Burden: Time Series Study in 272 Main Chinese Cities. Bmj (2018) 363:k4306. doi:10.1136/bmj.k4306
48. Xu, R, Shi, C, Wei, J, Lu, W, Li, Y, Liu, T, et al. Cause-Specific Cardiovascular Disease Mortality Attributable to Ambient Temperature: A Time-Stratified Case-Crossover Study in Jiangsu Province, China. Ecotoxicology Environ Saf (2022) 236:113498. doi:10.1016/j.ecoenv.2022.113498
49. Ferreira, LDCM, Nogueira, MC, Pereira, RVDB, de Farias, WCM, Rodrigues, MMDS, Teixeira, MTB, et al. Ambient Temperature and Mortality Due to Acute Myocardial Infarction in Brazil: An Ecological Study of Time-Series Analyses. Scientific Rep (2019) 9(1):13790–10. doi:10.1038/s41598-019-50235-8
50. Romani, SG, Royé, D, Sánchez Santos, L, and Figueiras, A. Impact of Extreme Temperatures on Ambulance Dispatches Due to Cardiovascular Causes in North-West Spain. Int J Environ Res Public Health (2020) 17(23):9001. doi:10.3390/ijerph17239001
51. Lam, HCY, Chan, JCN, Luk, AOY, Chan, EYY, and Goggins, WB. Short-Term Association Between Ambient Temperature and Acute Myocardial Infarction Hospitalizations for Diabetes Mellitus Patients: A Time Series Study. PLoS Med (2018) 15(7):e1002612. doi:10.1371/journal.pmed.1002612
52. Zhang, W, Du, G, Xiong, L, Liu, T, Zheng, Z, Yuan, Q, et al. Extreme Temperatures and Cardiovascular Mortality: Assessing Effect Modification by Subgroups in Ganzhou, China. Glob Health Action (2021) 14(1):1965305. doi:10.1080/16549716.2021.1965305
53. Li, N, Ma, J, Liu, F, Zhang, Y, Ma, P, Jin, Y, et al. Associations of Apparent Temperature With Acute Cardiac Events and Subtypes of Acute Coronary Syndromes in Beijing, China. Scientific Rep (2021) 11(1):15229–12. doi:10.1038/s41598-021-94738-9
54. de Moraes, SL, Almendra, R, and Barrozo, LV. Impact of Heat Waves and Cold Spells on Cause-Specific Mortality in the City of São Paulo, Brazil. Int J Hyg Environ Health (2022) 239:113861. doi:10.1016/j.ijheh.2021.113861
55. Lu, P, Zhao, Q, Xia, G, Xu, R, Hanna, L, Jiang, J, et al. Temporal Trends of the Association Between Ambient Temperature and Cardiovascular Mortality: A 17-Year Case-Crossover Study. Environ Res Lett (2021) 16(4):045004. doi:10.1088/1748-9326/abab33
56. Kranc, H, Novack, V, Shtein, A, Sherman, R, and Novack, L. Extreme Temperature and Out-of-Hospital-Cardiac-Arrest. Nationwide Study in a Hot Climate Country. Environ Health (2021) 20(1):38–13. doi:10.1186/s12940-021-00722-1
57. Chen, K, Breitner, S, Wolf, K, Hampel, R, Meisinger, C, Heier, M, et al. Temporal Variations in the Triggering of Myocardial Infarction by Air Temperature in Augsburg, Germany, 1987–2014. Eur Heart J (2019) 40(20):1600–8. doi:10.1093/eurheartj/ehz116
58. Li, M, Shaw, BA, Zhang, W, Vásquez, E, and Lin, S. Impact of Extremely Hot Days on Emergency Department Visits for Cardiovascular Disease Among Older Adults in New York State. Int J Environ Res Public Health (2019) 16(12):2119. doi:10.3390/ijerph16122119
59. Guo, YT, Bernard Goggins, W, Chan, EYY, and Ho, KF. Individual Socioeconomic Status as A Modifier of the Association Between High Ambient Temperature and Hospital Admissions: A Time Series Study in Hong Kong, 2010–2019. Environ Sci Pollut Res (2022) 29:67353–61. doi:10.1007/s11356-022-20512-7
60. Parliari, D, Cheristanidis, S, Giannaros, C, Keppas, SC, Papadogiannaki, S, de’Donato, F, et al. Short-Term Effects of Apparent Temperature on Cause-Specific Mortality in the Urban Area of Thessaloniki, Greece. Atmosphere (2022) 13(6):852. doi:10.3390/atmos13060852
61. Heo, S, Chen, C, Kim, H, Sabath, B, Dominici, F, Warren, JL, et al. Temporal Changes in Associations Between High Temperature and Hospitalizations by Greenspace: Analysis in the Medicare Population in 40 US Northeast Counties. Environ Int (2021) 156:106737. doi:10.1016/j.envint.2021.106737
62. Demoury, C, Aerts, R, Vandeninden, B, Van Schaeybroeck, B, and De Clercq, EM. Impact of Short-Term Exposure to Extreme Temperatures on Mortality: A Multi-City Study in Belgium. Int J Environ Res Public Health (2022) 19(7):3763. doi:10.3390/ijerph19073763
63. Hopp, S, Dominici, F, and Bobb, JF. Medical Diagnoses of Heat Wave-Related Hospital Admissions in Older Adults. Prev Med (2018) 110:81–5. doi:10.1016/j.ypmed.2018.02.001
64. Iranpour, S, Khodakarim, S, Shahsavani, A, Khosravi, A, and Etemad, K. Modification of the Effect of Ambient Air Temperature on Cardiovascular and Respiratory Mortality by Air Pollution in Ahvaz. Iran: Epidemiology and health (2020). p. 42.
65. Bi, X, Wu, C, Wang, C, Wang, Y, Wang, X, Song, C, et al. Impacts of Air Temperature and Its Extremes on Human Mortality in Shanghai, China. Urban Clim (2022) 41:101072. doi:10.1016/j.uclim.2021.101072
66. Liu, S, Chan, EYY, Goggins, WB, and Huang, Z. The Mortality Risk and Socioeconomic Vulnerability Associated With High and Low Temperature in Hong Kong. Int J Environ Res Public Health (2020) 17(19):7326. doi:10.3390/ijerph17197326
67. Yatim, ANM, Latif, MT, Sofwan, NM, Ahamad, F, Khan, MF, Mahiyuddin, WRW, et al. The Association Between Temperature and Cause-Specific Mortality in the Klang Valley, Malaysia. Environ Sci Pollut Res (2021) 28(42):60209–20. doi:10.1007/s11356-021-14962-8
68. Hu, K, Guo, Y, Hochrainer-Stigler, S, Liu, W, See, L, Yang, X, et al. Evidence for Urban–Rural Disparity in Temperature–Mortality Relationships in Zhejiang Province, China. Environ Health Perspect (2019) 127(3):037001. doi:10.1289/EHP3556
69. Royé, D, Codesido, R, Tobías, A, and Taracido, M. Heat Wave Intensity and Daily Mortality in Four of the Largest Cities of Spain. Environ Res (2020) 182:109027. doi:10.1016/j.envres.2019.109027
70. Iñiguez, C, Royé, D, and Tobías, A. Contrasting Patterns of Temperature Related Mortality and Hospitalization by Cardiovascular and Respiratory Diseases in 52 Spanish Cities. Environ Res (2021) 192:110191. doi:10.1016/j.envres.2020.110191
71. Moghadamnia, MT, Ardalan, A, Mesdaghinia, A, Naddafi, K, and Yekaninejad, MS. Association Between Apparent Temperature and Acute Coronary Syndrome Admission in Rasht, Iran. Heart Asia (2018) 10(2):e011068. doi:10.1136/heartasia-2018-011068
72. Can, G, Şahin, Ü, Sayılı, U, Dubé, M, Kara, B, Acar, HC, et al. Excess Mortality in Istanbul During Extreme Heat Waves Between 2013 and 2017. Int J Environ Res Public Health (2019) 16(22):4348. doi:10.3390/ijerph16224348
73. Alahmad, B, Shakarchi, AF, Khraishah, H, Alseaidan, M, Gasana, J, Al-Hemoud, A, et al. Extreme Temperatures and Mortality in Kuwait: Who Is Vulnerable? Sci Total Environ (2020) 732:139289. doi:10.1016/j.scitotenv.2020.139289
74. Fonseca-Rodríguez, O, Sheridan, SC, Lundevaller, EH, and Schumann, B. Hot and Cold Weather Based on the Spatial Synoptic Classification and Cause-Specific Mortality in Sweden: A Time-Stratified Case-Crossover Study. Int J biometeorology (2020) 64(9):1435–49. doi:10.1007/s00484-020-01921-0
75. Yu, X, Xia, L, Xiao, J, Zheng, J, Xu, N, Feng, X, et al. Association of Daily Mean Temperature and Temperature Variability With Onset Risks of Acute Aortic Dissection. J Am Heart Assoc (2021) 10(13):e020190. doi:10.1161/JAHA.120.020190
76. Jiang, S, Warren, JL, Scovronick, N, Moss, SE, Darrow, LA, Strickland, MJ, et al. Using Logic Regression to Characterize Extreme Heat Exposures and Their Health Associations: A Time-Series Study of Emergency Department Visits in Atlanta. BMC Med Res Methodol (2021) 21(1):87–9. doi:10.1186/s12874-021-01278-x
77. Pfeifer, K, Oudin Åström, D, Martinsone, Ž, Kaļužnaja, D, and Oudin, A. Evaluating Mortality Response Associated With Two Different Nordic Heat Warning Systems in Riga, Latvia. Int J Environ Res Public Health (2020) 17(21):7719. doi:10.3390/ijerph17217719
78. Lin, YK, Cheng, CP, Kim, H, and Wang, YC. Risk of Ambulance Services Associated With Ambient Temperature, Fine Particulate and Its Constituents. Scientific Rep (2021) 11(1):1651–10. doi:10.1038/s41598-021-81197-5
79. Schulte, F, Röösli, M, and Ragettli, MS. Heat-Related Cardiovascular Morbidity and Mortality in Switzerland: A Clinical Perspective. Swiss Med weekly (2021) 151(37):w30013. doi:10.4414/SMW.2021.w30013
80. Wang, YC, Lin, YK, Chen, YJ, Hung, SC, Zafirah, Y, and Sung, FC. Ambulance Services Associated With Extreme Temperatures and Fine Particles in a Subtropical Island. Scientific Rep (2020) 10(1):2855–11. doi:10.1038/s41598-020-59294-8
81. Oudin Åström, D, Åström, C, Forsberg, B, Vicedo-Cabrera, AM, Gasparrini, A, Oudin, A, et al. Heat Wave–Related Mortality in Sweden: A Case-Crossover Study Investigating Effect Modification by Neighbourhood Deprivation. Scand J Public Health (2020) 48(4):428–35. doi:10.1177/1403494818801615
82. Layton, JB, Li, W, Yuan, J, Gilman, JP, Horton, DB, and Setoguchi, S. Heatwaves, Medications, and Heat-Related Hospitalization in Older Medicare Beneficiaries With Chronic Conditions. PloS one (2020) 15(12):e0243665. doi:10.1371/journal.pone.0243665
83. Zhai, G, Tian, Y, Zhang, K, Qi, J, and Chai, G. The Effect of Apparent Temperature on Hospital Admissions for Cardiovascular Diseases in Rural Areas of Pingliang, China. Ann Agric Environ Med (2022) 29(2):281–6. doi:10.26444/aaem/147064
84. Scovronick, N, Sera, F, Acquaotta, F, Garzena, D, Fratianni, S, Wright, CY, et al. The Association Between Ambient Temperature and Mortality in South Africa: A Time-Series Analysis. Environ Res (2018) 161:229–35. doi:10.1016/j.envres.2017.11.001
85. Remigio, RV, Jiang, C, Raimann, J, Kotanko, P, Usvyat, L, Maddux, FW, et al. Association of Extreme Heat Events With Hospital Admission or Mortality Among Patients With End-Stage Renal Disease. JAMA Netw open (2019) 2(8):e198904. doi:10.1001/jamanetworkopen.2019.8904
86. Jiang, Y, Hu, J, Peng, L, Li, H, Ji, JS, Fang, W, et al. Non-Optimum Temperature Increases Risk and Burden of Acute Myocardial Infarction Onset: A Nationwide Case-Crossover Study at Hourly Level in 324 Chinese Cities. EclinicalMedicine (2022) 50:101501. doi:10.1016/j.eclinm.2022.101501
87. Guo, H, Du, P, Zhang, H, Zhou, Z, Zhao, M, Wang, J, et al. Time Series Study on the Effects of Daily Average Temperature on the Mortality From Respiratory Diseases and Circulatory Diseases: A Case Study in Mianyang City. BMC Public Health (2022) 22(1):1001–12. doi:10.1186/s12889-022-13384-6
88. Perčič, S, Kukec, A, Cegnar, T, and Hojs, A. Number of Heat Wave Deaths by Diagnosis, Sex, Age Groups, and Area, in Slovenia, 2015 vs. 2003. Int J Environ Res Public Health (2018) 15(1):173. doi:10.3390/ijerph15010173
89. Nairn, JR, and Fawcett, RJ. The Excess Heat Factor: A Metric for Heatwave Intensity and Its Use in Classifying Heatwave Severity. Int J Environ Res Public Health (2015) 12(1):227–53. doi:10.3390/ijerph120100227
90. Lawrence, MG. The Relationship Between Relative Humidity and the Dewpoint Temperature in Moist Air: A Simple Conversion and Applications. Bull Am Meteorol Soc (2005) 86(2):225–34. doi:10.1175/bams-86-2-225
91. Gasparrini, A, Armstrong, B, and Kenward, MG. Distributed Lag Non-Linear Models. Stat Med (2010) 29(21):2224–34. doi:10.1002/sim.3940
92. Maclure, M. The Case-Crossover Design: A Method for Studying Transient Effects on the Risk of Acute Events. Am J Epidemiol (1991) 133(2):144–53. doi:10.1093/oxfordjournals.aje.a115853
93. Davies, HTO, Crombie, IK, and Tavakoli, M. When Can Odds Ratios Mislead? Bmj (1998) 316(7136):989–91. doi:10.1136/bmj.316.7136.989
94. Zhang, J, and Kai, FY. What's the Relative Risk? a Method of Correcting the Odds Ratio in Cohort Studies of Common Outcomes. Jama (1998) 280(19):1690–1. doi:10.1001/jama.280.19.1690
95. Obradovich, N, Migliorini, R, Paulus, MP, and Rahwan, I. Empirical Evidence of Mental Health Risks Posed by Climate Change. Proc Natl Acad Sci (2018) 115(43):10953–8. doi:10.1073/pnas.1801528115
96. Pramanik, S, Punia, M, Yu, H, and Chakraborty, S. Is Dense or Sprawl Growth More Prone to Heat-Related Health Risks? Spatial Regression-Based Study in Delhi, India. Sustain Cities Soc (2022) 81:103808. doi:10.1016/j.scs.2022.103808
97. Moran, DS, Pandolf, KB, Shapiro, Y, Heled, Y, Shani, Y, Mathew, WT, et al. An Environmental Stress Index (ESI) as a Substitute for the Wet Bulb Globe Temperature (WBGT). J Therm Biol (2001) 26(4-5):427–31. doi:10.1016/s0306-4565(01)00055-9
98. Watts, JD, and Kalkstein, LS. The Development of a Warm-Weather Relative Stress Index for Environmental Applications. J Appl Meteorology Climatology (2004) 43(3):503–13. doi:10.1175/1520-0450(2004)043<0503:tdoawr>2.0.co;2
99. Becker, S. Bioclimatological Rating of Cities and Resorts in South Africa According to the Climate Index. Int J Climatology (2000) 20(12):1403–14. doi:10.1002/1097-0088(200010)20:12<1403:aid-joc539>3.0.co;2-v
100. Hamdi, M, Lachiver, G, and Michaud, F. A New Predictive Thermal Sensation Index of Human Response. Energy and Buildings (1999) 29(2):167–78. doi:10.1016/s0378-7788(98)00054-1
101. Malchaire, J, Piette, A, Kampmann, B, Mehnert, P, Gebhardt, HJ, Havenith, G, et al. Development and Validation of the Predicted Heat Strain Model. Ann Occup Hyg (2001) 45(2):123–35. doi:10.1093/annhyg/45.2.123
102. Nagano, K, and Horikoshi, T. Development of Outdoor Thermal Index Indicating Universal and Separate Effects on Human Thermal Comfort. Int J biometeorology (2011) 55(2):219–27. doi:10.1007/s00484-010-0327-z
103. Staiger, H, Laschewski, G, and Grätz, A. The Perceived Temperature–A Versatile Index for the Assessment of the Human Thermal Environment. Part A: Scientific Basics. Int J biometeorology (2012) 56(1):165–76. doi:10.1007/s00484-011-0409-6
104. Xu, Z, FitzGerald, G, Guo, Y, Jalaludin, B, and Tong, S. Impact of Heatwave on Mortality Under Different Heatwave Definitions: A Systematic Review and Meta-Analysis. Environ Int (2016) 89:193–203. doi:10.1016/j.envint.2016.02.007
105. Xu, Z, Cheng, J, Hu, W, and Tong, S. Heatwave and Health Events: A Systematic Evaluation of Different Temperature Indicators, Heatwave Intensities and Durations. Sci Total Environ (2018) 630:679–89. doi:10.1016/j.scitotenv.2018.02.268
106. Yang, TC, and Jensen, L. Climatic Conditions and Human Mortality: Spatial and Regional Variation in the United States. Popul Environ (2017) 38:261–85. doi:10.1007/s11111-016-0262-y
107. Choi, HM, Lee, W, Roye, D, Heo, S, Urban, A, Entezari, A, et al. Effect Modification of Greenness on the Association Between Heat and Mortality: A Multi-City Multi-Country Study. EBioMedicine (2022) 84:104251. doi:10.1016/j.ebiom.2022.104251
108. Kim, EJ, and Kim, H. Effect Modification of Individual-And Regional-Scale Characteristics on Heat Wave-Related Mortality Rates Between 2009 and 2012 in Seoul, South Korea. Sci Total Environ (2017) 595:141–8. doi:10.1016/j.scitotenv.2017.03.248
109. Madrigano, J, Mittleman, MA, Baccarelli, A, Goldberg, R, Melly, S, Von Klot, S, et al. Temperature, Myocardial Infarction, and Mortality: Effect Modification by Individual and Area-Level Characteristics. Epidemiology (Cambridge, Mass.) (2013) 24(3):439–46. doi:10.1097/EDE.0b013e3182878397
110. Medina-Ramón, M, Zanobetti, A, Cavanagh, DP, and Schwartz, J. Extreme Temperatures and Mortality: Assessing Effect Modification by Personal Characteristics and Specific Cause of Death in a Multi-City Case-Only Analysis. Environ Health Perspect (2006) 114(9):1331–6. doi:10.1289/ehp.9074
111. O’Neill, MS, Zanobetti, A, and Schwartz, J. Modifiers of the Temperature and Mortality Association in Seven US Cities. Am J Epidemiol (2003) 157(12):1074–82. doi:10.1093/aje/kwg096
112. Yang, J, Ou, CQ, Ding, Y, Zhou, YX, and Chen, PY. Daily Temperature and Mortality: A Study of Distributed Lag Non-Linear Effect and Effect Modification in Guangzhou. Environ Health (2012) 11(1):63–9. doi:10.1186/1476-069X-11-63
113. Lavados, PM, Olavarría, VV, and Hoffmeister, L. Ambient Temperature and Stroke Risk: Evidence Supporting A Short-Term Effect at A Population Level From Acute Environmental Exposures. Stroke (2018) 49(1):255–61. doi:10.1161/STROKEAHA.117.017838
114. Lian, H, Ruan, Y, Liang, R, Liu, X, and Fan, Z. Short-Term Effect of Ambient Temperature and the Risk of Stroke: A Systematic Review and Meta-Analysis. Int J Environ Res Public Health (2015) 12(8):9068–88. doi:10.3390/ijerph120809068
115. Kyobutungi, C, Grau, A, Stieglbauer, G, and Becher, H. Absolute Temperature, Temperature Changes and Stroke Risk: A Case-Crossover Study. Eur J Epidemiol (2005) 20(8):693–8. doi:10.1007/s10654-005-0703-x
116. Nawrot, TS, Staessen, JA, Fagard, RH, Bortel, LMV, and Struijker-Boudier, HA. Endothelial Function and Outdoor Temperature. Eur J Epidemiol (2005) 20(5):407–10. doi:10.1007/s10654-005-1068-x
117. Sankoff, J. Heat Illnesses: A Hot Topic in the Setting of Global Climate Change. Aust Fam Physician (2015) 44(1/2):22–6.
118. Bhaskaran, K, Armstrong, B, Hajat, S, Haines, A, Wilkinson, P, and Smeeth, L. Heat and Risk of Myocardial Infarction: Hourly Level Case-Crossover Analysis of Minap Database. Bmj (2012) 345:e8050. doi:10.1136/bmj.e8050
119. Abrignani, MG, Lombardo, A, Braschi, A, Renda, N, and Abrignani, V. Climatic Influences on Cardiovascular Diseases. World J Cardiol (2022) 14(3):152–69. doi:10.4330/wjc.v14.i3.152
120. Cheng, J, Xu, Z, Bambrick, H, Prescott, V, Wang, N, Zhang, Y, et al. Cardiorespiratory Effects of Heatwaves: A Systematic Review and Meta-Analysis of Global Epidemiological Evidence. Environ Res (2019) 177:108610. doi:10.1016/j.envres.2019.108610
Keywords: heat, extreme temperature, cardiovascular health, mortality, morbidity
Citation: Nawaro J, Gianquintieri L, Pagliosa A, Sechi GM and Caiani EG (2023) Heatwave Definition and Impact on Cardiovascular Health: A Systematic Review. Public Health Rev 44:1606266. doi: 10.3389/phrs.2023.1606266
Received: 01 June 2023; Accepted: 29 September 2023;
Published: 16 October 2023.
Edited by:
Ana Ribeiro, University Porto, PortugalReviewed by:
Ana Monteiro, University of Porto, PortugalMónica Rodrigues, University of Coimbra, Portugal
Copyright © 2023 Nawaro, Gianquintieri, Pagliosa, Sechi and Caiani. This is an open-access article distributed under the terms of the Creative Commons Attribution License (CC BY). The use, distribution or reproduction in other forums is permitted, provided the original author(s) and the copyright owner(s) are credited and that the original publication in this journal is cited, in accordance with accepted academic practice. No use, distribution or reproduction is permitted which does not comply with these terms.
PHR is edited by the Swiss School of Public Health (SSPH+) in a partnership with the Association of Schools of Public Health of the European Region (ASPHER)+
*Correspondence: Enrico Gianluca Caiani, enrico.caiani@polimi.it
This Systematic Review is part of the PHR Special Issue “Neighbourhood Influences on Population Health”