- 1Laboratoire MESuRS, Conservatoire National des Arts et Métiers, Paris, France
- 2Unité PACRI, Institut Pasteur, Conservatoire National des Arts et Métiers, Paris, France
- 3Centre International de Recherche sur l’Environnement et le Développement (CIRED), Centre National de la Recherche Scientifique, (CNRS), Nogent-sur-Marne, France
- 4Centre for Environmental Policy, MRC Centre for Environment and Health, Imperial College London, London, United Kingdom
- 5Association NegaWatt, Alixan, France
- 6MRC Centre for Global Infectious Disease Analysis, Department of Infectious Disease Epidemiology, Imperial College London, London, United Kingdom
Objectives: Energy transition scenarios are prospective outlooks describing combinations of changes in socio-economic systems that are compatible with climate targets. These changes could have important health co-benefits. We aimed to quantify the health benefits of physical activity caused by active transportation on all-cause mortality in the French negaWatt scenario over the 2021–2050 period.
Methods; Relying on a health impact assessment framework, we quantified the health benefits of increased walking, cycling and E-biking projected in the negaWatt scenario. The negaWatt scenario assumes increases of walking and cycling volumes of +11% and +612%, respectively, over the study period.
Results: As compared to a scenario with no increase in volume of active travel, we quantified that the negaWatt scenario would prevent 9,797 annual premature deaths in 2045 and translate into a 3-month increase in life expectancy in the general population. These health gains would generate €34 billion of economic benefits from 2045 onwards.
Conclusion: Increased physical activity implied in the negaWatt transition scenario would generate substantial public health benefits, which are comparable to the gain expected by large scale health prevention interventions.
Introduction
The health impacts of climate change have recently become more prominent features of climate change discussions, as exemplified by the World Health Organization active engagement in COP26 [1] and the extraordinary joint publication across 200 health journals of a call for urgent climate action to protect health [2]. To mitigate the effects of climate change for current and future generations, countries need to cut their greenhouse gas emissions (GHG) and reach the global target of net zero emissions by the middle of the 21st century [3]. These efforts could potentially result in important short-term health benefits, especially by improving air quality, housing, and diets, and increasing physical activity [4]. Demonstrating health co-benefits of climate mitigation actions through a rigorous assessment could help make the case for transformative strategies and play a key role in increasing adhesion and commitment of people and their governments in reducing GHG emissions [5, 6].
Government and civil societies can contribute important efforts in exploring and understanding desirable pathways toward carbon neutrality. In France, governmental agencies, non-profit organizations, and other groups of qualified experts developed several carbon neutrality scenarios. These transition scenarios describe various combination of changes in socio-economic systems that are compatible with achieving a climate target. Based on a transdisciplinary approach, these scenarios provide explicit details on the scope and distribution of reduction efforts and on the role of the different levers of change, whether political, technological or behavioural [7, 8].
In Europe, transport represents the second sector contributing the most to GHG emissions [9]. In France in particular, transportation contributes to 31% of the national GHG emissions, making it the highest emitter. With about half of these emissions attributable to individual motorized vehicles, modal shifts towards low-carbon transport modes such as public or active transportation constitute an important element for national decarbonisation pathways [10].
There is compelling evidence that sedentary lifestyles cause a large health burden, especially in high-income countries, and that increasing physical activity could provide important public health benefits [11, 12]. Specifically, the potential for active transport modes such as cycling and biking to alleviate mortality and morbidity are now well established [13, 14]. Therefore, climate change mitigation actions resulting in increasing active transportation may carry important public health benefits [4, 15, 16]. However, these benefits have not been quantified, yet, within the framework of a transition scenario describing detailed and credible societal transformations toward carbon neutrality.
In this study, we aimed at quantifying the health benefits of physical activity generated by active transportation on all-cause mortality in a French transition scenario over the 2021–2050 period. To do so, we relied on the framework of health impact assessment and followed recent guidelines for modelling and reporting health effects of climate change mitigation actions [17, 18].
Methods
The negaWatt Scenario
The negaWatt association has been developing energy transition scenarios for metropolitan France since 2003. These scenarios offer coherence in energy flows and a high level of sectoral detail while providing explicit data on the main physical determinants of energy consumption (heated surfaces, km-passengers) with a yearly time resolution. The latest negaWatt scenario was released in 2021.
In order to reach net zero emissions in 2050, the negaWatt approach combines energy sufficiency (favouring behaviours and activities that are intrinsically low in energy use, at individual and collective levels), energy efficiency, and renewable sources [19]. For the transportation sector, the scenario classifies trips based on their motives (commuting, occasional leisure, professional, etc.), lengths, and locations (urban centres, rural areas, etc.).
The negaWatt scenario activates several levers to decarbonize the transportation sector [20]. First, the scenario assumes an overall decrease in transportation demand, mostly driven by a decrease in long-distance trips, an increase in teleworking, and an overall relocation of activities. Then, ambitious fiscal measures would internalize all externalities of transportation (CO2, air pollution, noise) while accounting for issues of social and territorial inequalities. These measures would allow important investments in public and active transportation infrastructures, such as railways and bike lanes. These would in turn yield to large modal shifts from individual motorized vehicles toward public and active transportation, especially for short trips. Motorized vehicles would remain the most common transport mode in terms of km-passengers (Supplementary Datasheet S2), but decarbonisation is achieved assuming a reduction in the weight of cars, a higher vehicle occupancy rate (carsharing), and lastly a shift toward renewable energy, such as electricity and biogas.
The evolution of walking and cycling mileage projected in the negaWatt scenario for 2021–2050 is presented in Figure 1. The weekly number of kilometres walked per inhabitant increases slightly and regularly, with an overall increase of +11% over the study period. The distance cycled increases most dramatically between 2021 and 2040, from 2.4 km inh−1 week−1 to 17.1 km inh−1 week−1 (+612%), it peaks at 17.5 km inh−1 w−1 in 2045, and then decreases back to 17.1 km inh−1 week−1 in 2050. This increase is distributed between classical bikes and E-bikes, with an overall contribution of E-bikes increasing from 3.3% of the kilometres cycled in 2021 to 70% in 2040, this later proportion being kept constant over 2040–2050. The increase in distances cycled together with the relative decrease in the contribution of classical bikes explains the inverse U-shape peaking in 2032 that is observed for classical bike. As a comparison, the average distance travelled by car (regardless of the energy used) decreases by nearly 40% between 2021 and 2050 (from approximatively 12,000 to 7,500 km inh−1 y−1).
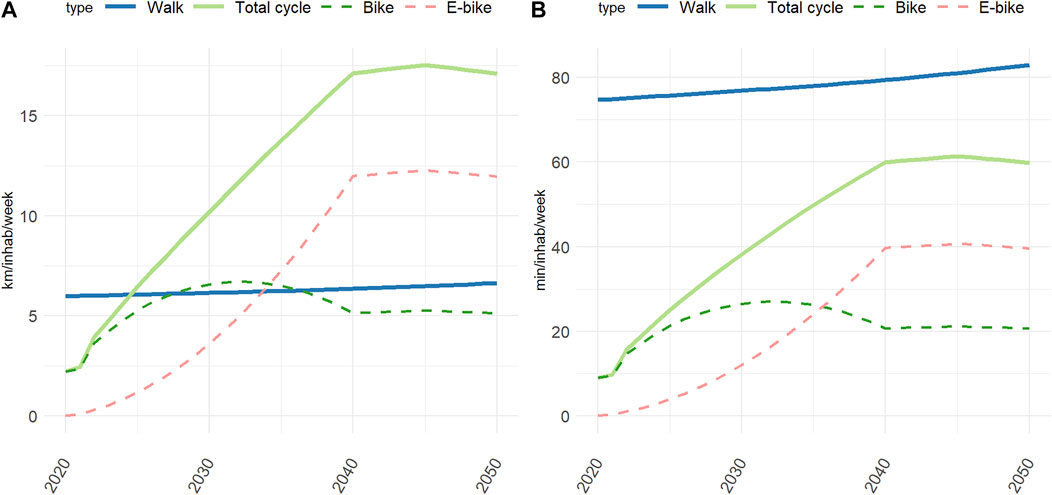
FIGURE 1. Evolution of weekly walking and cycling mileage (A) and duration (B) (negaWatt scenario, France, 2020–2050). Calculation of duration assumed an average speed of 4.8 km h−1, 14.9 km h−1, 18.1 km h−1 for walking, cycling and E-biking, respectively.
Age-Distribution of Cycling
There is currently a scarcity of reliable data regarding the frequency, volume (mileage) and age-distribution of active transportation in France. Results of the most recent nationally representative surveys have been released in early 2022, but at the time we conducted this analysis, the most recent nationally representative data was collected in 2008 and documented a biking mileage that was largely concentrated in young men aged <25 years [21]. Investment in active transportation promotion and infrastructure, as well as technological development (notably E-bikes) are expected to have flattened this distribution. Moreover, a large increase in active transportation such as the one projected in the negaWatt scenario implies that all age groups would be affected. We thus used the age-distribution of walking and cycling mileage reported from Denmark, one of the countries with the highest cycling level in Europe, as a target distribution to allocate the yearly global mileage projected by the negaWatt scenario across ages. The Danish age-distribution of active transportations mileage for 2016–2019 was obtained from the Danish National Travel Survey [22]. We assumed the overall relative increase in walking and cycling to affect all ages homogeneously. However, for cycling, we attributed the total number of kilometres cycled between E-bike and classical bike differentially across age group in order to both reproduce: i) the 6.7 years difference observed in the mean age of E-bikers and cyclists reported in a recent multi-country study [23], and ii) the time-changing relative contribution of E-bike to the total cycling mileage assumed in the negaWatt scenario. Further details and formulas are provided in Supplementary Datasheet S1. For the reference (or business as usual) scenario we assumed the same relative age-distribution of active travel as detailed above, but the total mileage of active travel was assumed to be the same as in our base year, 2021.
Health Impact Assessment
The geographic scope of the study is metropolitan France and the time-period of the analysis is 2021–2050. Projections of demographic data for population size, age distribution and mortality rates used in the negaWatt scenario and in the HIA were obtained from the National Institute of Statistics and Economic Studies [24].
We relied on the framework of the Health Economic Assessment Tool (HEAT) developed by the World Health Organization to estimate the number of deaths prevented by active transportation [25]. Based on the meta-analysis by Kelly et al for the reduction in all-cause mortality from walking and cycling [14], we assumed a reduction by 10% in all-cause mortality (95% confidence interval, CI: 6%–13%) for a weekly exposure of 100 min of cycling, and a reduction of 11% (95% CI: 4%–17%) for a weekly exposure of 168 min of walking. Both of these reference volumes represent a metabolic equivalent of task hours per week of 11.25 MET hour week−1, independently of other physical activity, which correspond to the level of physical activity recommended by international guidelines [26]. As the meta-analysis by Kelly et al considered all-cause mortality, negative sides of active transportation via road safety and increased exposure to air pollution were controlled for. We assumed that the physical activity required for an E-bike was 90% of the one required for a classical bike [16]. Risk reduction estimates were scaled to the yearly level of the corresponding active travel using a linear dose-response function (DRF) which was capped at a maximum level of risk reduction (45% for cycling and 30% for walking) [14]. In the main analysis, the age range considered for a reduction of mortality attributable to physical activity increase was 20–84 years. Younger and older ages were disregarded because the evidence for the health effects of physical activity was not as large as that for the 20–84 years.
Yearly distances travelled by cycling, E-bike or walking were converted into times of exposure considering an average speed of 14.9 km h−1, 18.1 km h−1 and 4.8 km h−1, respectively [16, 25]. We assumed the same values for E-bike speed and E-bike-to-classical-bike MET ratio as those recently identified in the literature by Egiguren et al. [16]. The scaled values of risk reduction were then applied to the projections of the age-specific (1-year age bands) mortality rate in both (ie. negaWatt and reference) scenarios for each year from 2022 to 2050. This allowed to estimate, for both scenarios, the yearly number of premature deaths averted by active transportation for each age. Based on the yearly projected value of life-expectancy, we also calculated the years of life lost (YLL) averted by active transportation associated with each scenario and for each travel mode, age and year. The health impact of the increase in active transportation in the negaWatt scenario as compared to the reference scenario was estimated as the difference between the number of deaths averted and YLL in the negaWatt and the reference scenarios. We lastly used age- and year-specific death rates in each scenario to estimate the increase in life expectancy due to active transportation in the negaWatt scenario.
Health Economics Evaluation
To estimate the economic health benefits of active transportation implied by the negaWatt scenario, we used the standard value of statistical life year (VSLY) that was recommended in 2013 in France for the socioeconomic evaluation of public investments [27]. The recommended VSLY was 139k€ in 2020, and the values recommended for projections account for economic growth (publicly available here: https://www.strategie.gouv.fr/sites/strategie.gouv.fr/files/atoms/files/20181214_complement_b_valeurs_tutelaires.xlsx). All amounts were expressed in Euros 2020.
Sensitivity Analysis
We conducted several alternative analyses to assess the sensitivity of our results to specific parameters values or assumptions. The various alternative assessments we conducted considered: i) no age difference between classical bike and E-bike users; ii) a ratio of MET.hour of 0.78 for the physical activity required for an E-bike vs. a classical bike, as assumed by Bouscasse et al. [28]; iii) an age limit of 75 years above which physical activity doesn’t decrease the mortality risk; iv) a reduction by 19% (95% CI: 9%–29%) of the risk of mortality for an exposure of 11.25 MET hour week−1, as recently documented by the meta-analysis by Zhao et al. [29], which we also used to scale the risk reduction for E-bike.
All estimates were provided together with uncertainty intervals (UI) calculated using the lower and upper bounds of the 95% CI reported for the reduction of the mortality risk for each active transportation mode. Analyses were conducted using the R software version 4.1.2. All codes are publicly available at: https://github.com/pbarban/HIA_Transport_Transition.
Results
The distribution of weekly active transportation durations across age groups is presented in Figure 2. Based on the shape of the distribution observed in Denmark, walking is distributed fairly homogeneously across age groups. The total cycling distribution is more heterogeneous, with the 20–29 years-old group contributing the most to the global mileage. Although the increase over time of walking and cycling is assumed to be proportional across each age group, the age-specific evolution is different when considering classical vs. E-bike. While E-bike exposure is projected to be low across all age groups in 2025, we assume the increases over time to be proportionally higher in the older vs. younger age groups in comparison with the increase in classic cycling. For instance, in 2045, 58% of distances cycled is attributed to E-bike for the 15–19 years-old group, and 81% among the 65–69 years-old group.
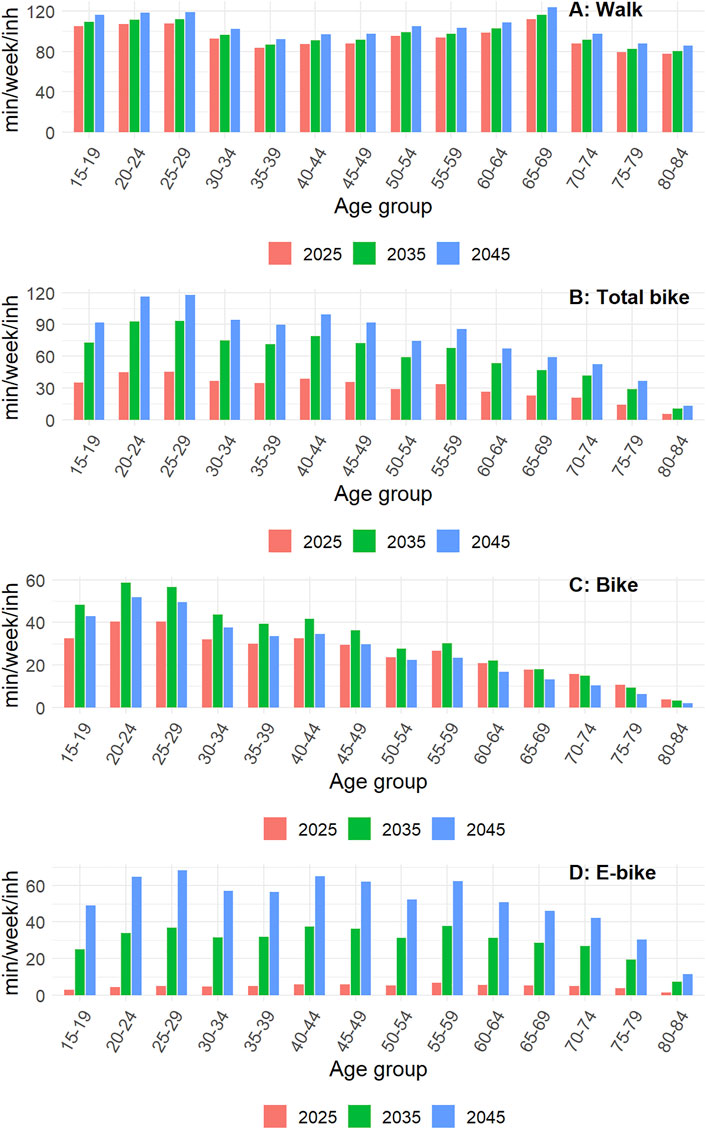
FIGURE 2. Evolution of weekly walking and cycling duration by age (negaWatt scenario, France, 2020–2050).
Health Impact Assessment
The health impact estimated for active transportation over the 2021–2050 period are presented in Figure 3. As compared to a reference scenario in which walking and cycling mileage were maintained constant at their 2021 levels, the increased use of active transportation could prevent a cumulative 213,000 (UI: 122,000 −283,000) premature deaths across France from 2021 to 2050, with a yearly impact peaking at 9,825 [5,538–13,143] premature deaths prevented in 2045. The contribution of each transport mode varied across time (Figure 4). Over the whole study period, relatively to the cumulative number of deaths prevented, walking contributed to 11.3%, classical bike to 22.7%, and E-bike to 66.0%; these contributions were 8.7%, 27.9% and 63.4%, respectively, relatively to the cumulative number of YLL prevented.
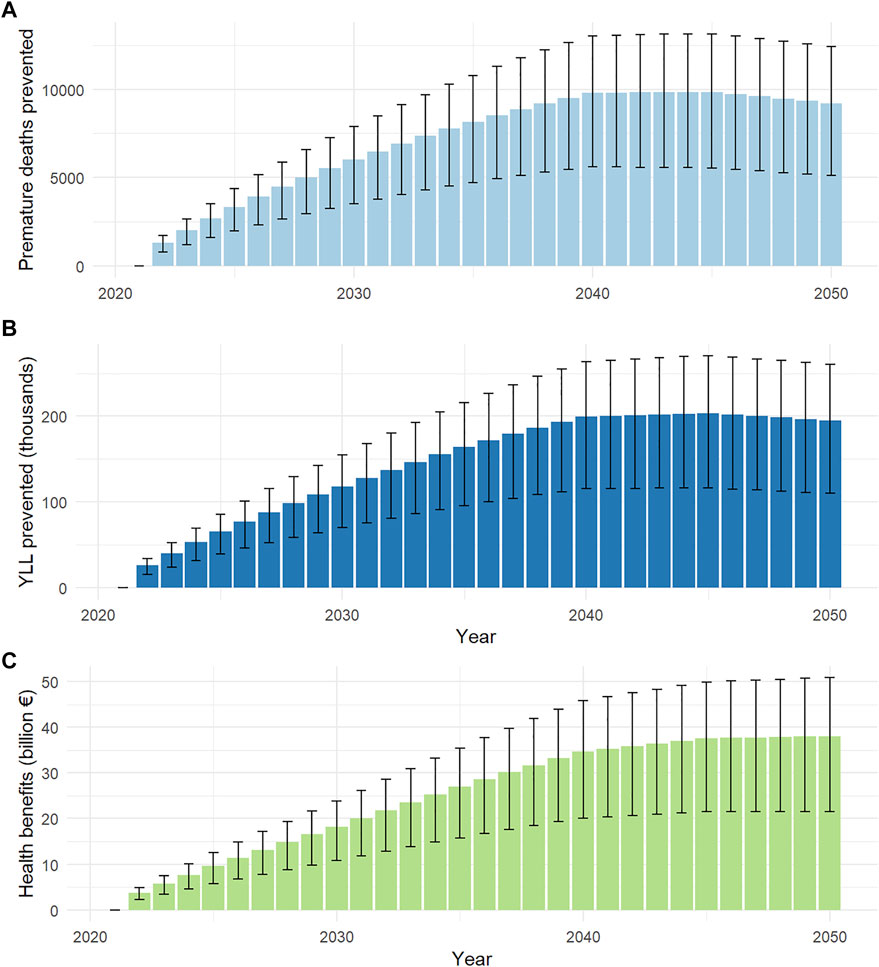
FIGURE 3. Premature deaths prevented (A), years of life lost (YLL) prevented (B) and monetised health benefits (C) of active transportation (negaWatt scenario, France, 2020–2050). Health benefits are calculated based on the value of statistical life year.
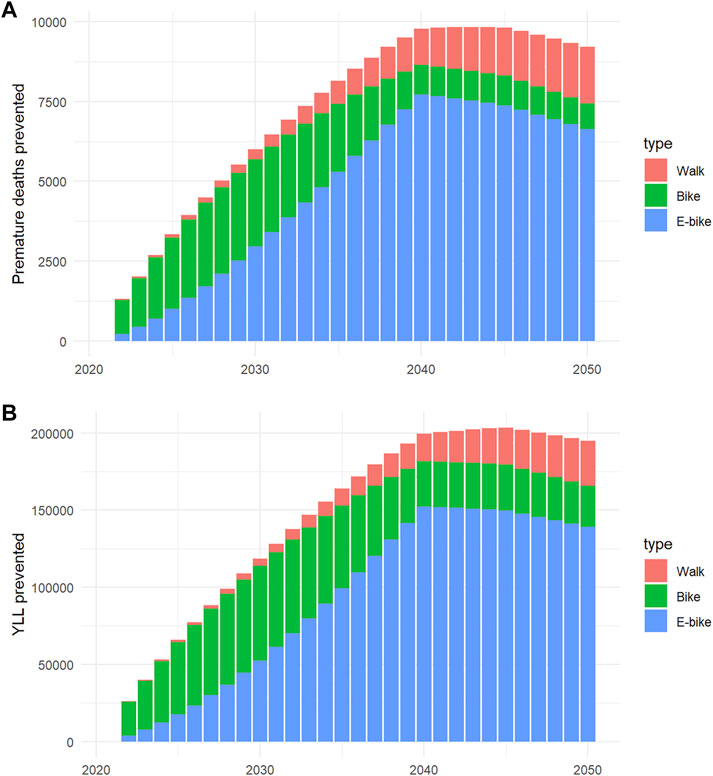
FIGURE 4. Premature deaths (A) and years of life lost (YLL, (B)) prevented per type of active transportation (negaWatt scenario, France, 2020–2050).
Deaths prevented were largely distributed among older age groups, especially among the 70–79 years-old group. For instance, in 2045, this age group concentrated 37.2% of premature deaths averted despite their contribution to only 9.1% of all km travelled, regardless of travel mode. In contrast, the distribution of YLL prevented was more widely distributed across age groups, and its mode was the 55–59 years-old group (Figure 5).
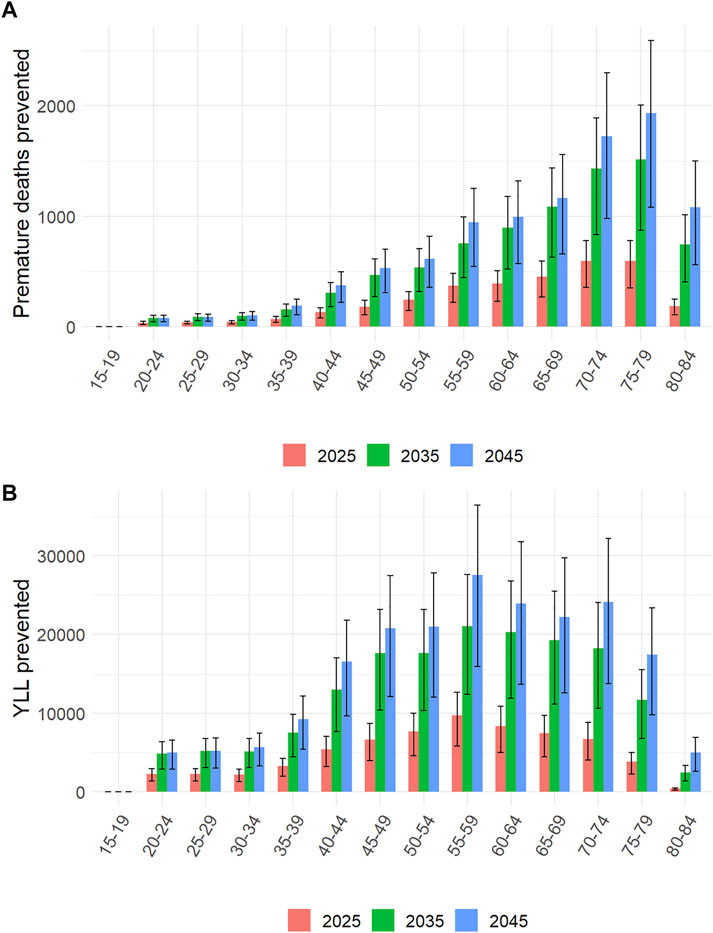
FIGURE 5. Contribution of the different age groups to premature deaths (A) and years of life lost (YLL, (B)) prevented by active transportation (negaWatt scenario, 2020–2050).
By considering age at death, we assessed that increased active transportation could prevent a cumulative 4.34 (UI: 2.52 – 5.74) millions of YLL over the study period), with a yearly amount peaking at 204 (UI: 116–271) thousands YLL prevented in 2045. We further estimated that the increased use of active transportation implied by the negaWatt scenario could result in a life expectancy gain of 3.39 (UI: 1.93–4.53) months over the general population in 2045 (Supplementary Datasheet S3).
Based on recommended values of a statistical life year, we estimated the corresponding total economic health benefits that progressively increases to peak at €37.6 (UI: 21.5–49.9) billion in 2045, then plateauing after 2045. This represented a cumulative economic health benefits of €749 (UI: 433–990) billion over the 2021–2050 time period.
Sensitivity Analysis
Sensitivity of our results depending on the choice of the parameters are presented in Table 1. Assuming that there are no differences in age between bike vs. E-bike users, or considering an alternative lower value of the E-bike-to-classical-bike MET ratio did not impact massively our results. Considering an age limit below 75 years (vs. 85 years in the main analysis) to consider health benefits for physical activity decreased the estimated yearly and cumulative death prevented by approximatively 35%. However, the impact on the monetized health benefits was more limited (an approximated 10% decrease). Indeed, monetized health benefits calculation were based on YLL, and deaths prevented between 75 and 85 years are not those contributing the most to the overall YLL prevented by physical activity. Lastly, the parameters that appeared to have the largest impact on our results was the RR considered for health impact of biking (and consequently for E-bike as we maintained the same ratio for physical activity between bike and E-bike as in the main analysis). Using the more favourable value recently reported in the meta-analysis by Zhao et al [29] leads to approximately doubling the estimates of health benefits, be it in terms of death prevented, YLL prevented, or life expectancy gain.
Discussion
In this study, we estimated the health impacts of active transportation as projected in the negaWatt transition scenario, France. We estimate that increased use of walking and cycling could prevent up to 10,000 (between 5,000 and 13,000, approximatively) premature deaths and 190,000 YLL (between 105,000 and 250,000, approximatively) yearly in 2045. The uncertainty interval of these estimates reflects mostly the uncertainty surrounding the dose-response function linking physical activity and mortality. The health benefits could result in a life expectancy gain of about 3 months in 2045 for the general population. The health economic benefits generated by this burden alleviation could represent a cumulative €696 (between 403 and 919) billion over the 2022–2050 time period, and an approximate €35 billion annually from 2045 onwards. To our knowledge, this study constitutes the first one to assess the health co-benefits of a credible pathway to net zero emission at the level of a country.
The negaWatt scenario we assessed assumes several mobility behavioural changes. First, it assumes a small but sustained increase in walking over the study period (+11%). This increase could be achieved by modal shifts from motorized vehicles to walking for short trips, or more broadly to public transportation, as this mode implies a certain amount of walking [30]. The projected increase in cycling in the scenario is much larger (+612%). As France lags behind many countries in current levels of cycling [31], the highest value of cycling volume projected for 2045 (62 min week−1) in our scenario was still not dramatically higher than current average cycling in Denmark (45 min week−1) and remains below the average cycling duration in the Netherlands (74 min week−1 in 2010–2013) [22, 32]. Moreover, this overall increase in cycling was mostly driven by E-bikes, projected to represent up to 70% of all kilometres cycled from 2040 onwards. Indeed, according to recent studies E-bikes constitute a promising transportation mode for many, especially for older adults, allowing longer trips than classical bikes and thus having a fair potential for car trips substitutions [23, 33, 34].
Cycling, either classical or E-bike, represented the largest health benefits in our study. Our results were therefore sensitive to the DRF chosen to quantify reductions of all-cause mortality by increased cycling. Our main analysis relied on a DRF reported in a meta-analysis synthetizing the results 7 prospective studies and widely used in cycling-related HIAs [25, 28, 32, 35]. The more favourable DRF documented by the recent meta-analysis by Zhao et al used in our sensitivity analysis led to a doubling of the health impact estimates. Our approach built on the HEAT framework by explicitly accounting for age [25]. In a conservative assumption, HEAT assumes no reduction in mortality due to physical activity beyond 65 years, while our main analysis assumed such a preventive effect up to 84 years. Indeed, both meta-analyses used in our assessment included studies providing data beyond age 65. Moreover, there is increasing evidence that regular physical activity in older people can reduce the risk of falls and fractures, and thus may reduce mortality [36].
Several studies have previously assessed the health benefits of increased active transportation for France. Praznoczy estimated in 2012 that physical activity induced by a shift of 20% of modal share toward biking could prevent ∼3,000 premature deaths in 2020 in Ile-de-France (12.2 million inh., ∼20% of the population considered here) [37]. More recently, Egiguren et al estimated that a high-biking scenario could prevent ∼2,600 annual premature deaths in France in 2030 [16]. However, direct comparisons with our results are not straightforward as these studies relied on different geographical scopes and on varying assumptions, especially regarding the evolution of cycling mileage and modal share. In addition to the existing literature, our study highlights the potential for active transportation promotion policies to yield important public health benefits in the medium and long term [38]. We estimated that active transportation implied by the negaWatt scenario may prevent up to 10,000 deaths annually from 2045 onwards. For comparison, efforts in road safety made in France over the past 10 years may have prevented about 1,500 deaths yearly; and public health policies aiming at decreasing alcohol consumption by 20% in France would result in approximatively 7,000 deaths prevented a year [39, 40].
This assessment carries various limitations. On the one hand, we estimated the health impact of the negaWatt scenario as compared to a business-as-usual scenario that considered the level of active transportation constant from 2021 onward. Disregarding possible increases in cycling, as those having been reported in many French cities may have led to overestimating health impacts [41]. However, these recent increases have been partly linked to conjectural factors, such as the Covid-19 impact on public transportation, and one can question their durability in the absence of dedicated policies. On the other hand, the negaWatt scenario assumes a strong and continuous increase of cycling between 2021 and 2040. This increase could be partly hampered by increasing frequency and duration of heat waves that are predicted in the near and medium term due to climate change [42]. Our study assessed the health impact of active transportation for those exposed to these modes only. Pedestrian and bike-users are also exposed to negative health outcomes, such as increased risk of traffic injury and increased exposure to air pollution. The DRF for all-cause mortality we used captures both positive and negative effects of cycling and walking. The present analysis therefore implicitly accounted for the detrimental effects of walking and cycling. However, active transportation also carries altruistic health benefits beyond the groups of pedestrians and bike-users, such as reduced overall air or noise pollution. These altruistic benefits are not included in the present analysis, mostly due to methodological issues. The health benefits we document here may thus have been underestimated. Indeed, In a multi-country analysis, Hamilton et al estimated that the benefits resulting from improved air quality implied by the Paris Agreement may be of the same order of magnitude than those resulting from active travel (although this ratio varied largely across countries) [4].
This study documents that physical activity due to increased active transportation projected in a transition scenario would generate substantial public health benefits, which may be comparable to the gains expected by large-scale health prevention interventions. The present study shows that these health gains may translate into important monetised health benefits on the short term, thus providing further arguments in favour of ambitious climate mitigation investment and policies. The fact that an energy transition scenario assuming increasing active transportation may generate health benefits is itself unsurprising and expected. However, the rigorous quantification of such benefits offers more detailed elements, especially to weight the costs of policies promoting active transportation against their benefits. Furthermore, several levers may be activated to decarbonize the transportation sector. For instance, some other French transition scenarios rely much more widely on the electrification of the vehicle fleet than the negaWatt scenario does [43]. The present study thus suggests that betting on vehicle electrification only may miss the opportunity of large public health benefits generated by increased physical activity.
The primary novelty of this study is that our estimates are based on a detailed and internally consistent scenario detailing an explicit pathway toward carbon neutrality at the scale of a country. The negaWatt association has developed these energy scenarios since 2003 and their influence on government policies has grown since then. Government agencies such as the French Agency for ecological transition have now produced energy scenarios quite similar to the negaWatt scenarios, in particular with regards to growth in active transportation. Previous similar health impact assessments of ambitious climate targets consisted in evaluating co-benefits of these targets once they were reached, with no consideration of the pathway [4, 15]. This study is a demonstration that realistic scenarios to reach net zero for climate change purposes can generate benefits far beyond reductions in GHG only. This is the type of evidence that can help create alliances across sectors and garner support for much needed transitions [6]. In other words, this contribution may help engage the climate change and public health scientific communities, along with the broader civic society, to promote health-enhancing pathways to net zero emissions.
Author Contributions
PB, PQ, and KJ conceived the original idea. SC complied input data. PB and KJ conducted the analysis and produced output figures and tables. PB, PQ, and KJ interpreted the results. PB and KJ wrote the first draft of the article. All authors provided critical feedback and helped shape the research, analysis and manuscript.
Conflict of Interest
The authors declare that the research was conducted in the absence of any commercial or financial relationships that could be construed as a potential conflict of interest.
Acknowledgments
We are thankful to Aurélien Bigo (ADEME, Angers, and Chair Energy and Prosperity, Paris, France) for useful discussions; and to Laura Temime (Laboratoire MESuRS, Cnam, Paris, France) and Julien Reygner (CERMICS – Ecole des Ponts ParisTech, Marne la Vallée, France) for insights on mathematical aspects related to this work. This work has been firstly published on the medRxiv pre-print server [44].
Supplementary Material
The Supplementary Material for this article can be found online at: https://www.ssph-journal.org/articles/10.3389/ijph.2022.1605012/full#supplementary-material
References
1.World Health Organization. COP26 Health Programme (2021). Available at: https://www.who.int/initiatives/cop26-health-programme (Accessed June 23, 2022).
2. Atwoli, L, Baqui, AH, Benfield, T, Bosurgi, R, Godlee, F, Hancocks, S, et al. Call for Emergency Action to Limit Global Temperature Increases, Restore Biodiversity, and Protect Health. BMJ (2021) 374:n1734. doi:10.1136/bmj.n1734
3. Rogelj, J, Shindell, D, Jiang, K, Fifita, S, Forster, P, Ginzburg, V, et al. Mitigation Pathways Compatible with 1.5°C in the Context of Sustainable Development. Global Warming of 15 °C (2018). p. 93–174.
4. Hamilton, I, Kennard, H, McGushin, A, Höglund-Isaksson, L, Kiesewetter, G, Lott, M, et al. The Public Health Implications of the Paris Agreement: a Modelling Study. Lancet Planet Health (2021) 5(2):e74–83. doi:10.1016/s2542-5196(20)30249-7
5. Flahault, A, Schütte, S, Guégan, JF, Pascal, M, and Barouki, R. Health Can Help Saving Negotiation on Climate Change. Lancet (2015) 385(9985):e49–50. doi:10.1016/S0140-6736(15)60866-2
6. Negev, M, Zea-Reyes, L, Caputo, L, Weinmayr, G, Potter, C, and de Nazelle, A. Barriers and Enablers for Integrating Public Health Cobenefits in Urban Climate Policy. Annu Rev Public Health (2021) 43:255. doi:10.1146/annurev-publhealth-052020-010820
7. Guivarch, C, Lempert, R, and Trutnevyte, E. Scenario Techniques for Energy and Environmental Research: An Overview of Recent Developments to Broaden the Capacity to deal with Complexity and Uncertainty. Environ Model Softw (2017) 97:201–10. doi:10.1016/j.envsoft.2017.07.017
8. Elsawah, S, Hamilton, SH, Jakeman, AJ, Rothman, D, Schweizer, V, Trutnevyte, E, et al. Scenario Processes for Socio-Environmental Systems Analysis of Futures: A Review of Recent Efforts and a Salient Research Agenda for Supporting Decision Making. Sci Total Environ (2020) 729:138393. doi:10.1016/j.scitotenv.2020.138393
9.European Environment Agency. Annual European Union Greenhouse Gas Inventory 1990–2018 and Inventory Report 2020. Copenhagen, Denmark: European Environment Agency (2020). Available at: https://www.eea.europa.eu//publications/european-union-greenhouse-gas-inventory-2020 (Accessed January 14, 2022).
10.The High Council on Climate (HCC). Renforcer L’atténuation, Engager L’adaptation [Reinforce Mitigation, Engage Adaptation]. Paris, France (2021). Available at: https://www.hautconseilclimat.fr/publications/rapport-annuel-2021-renforcer-lattenuation-engager-ladaptation/ (Accessed June 23, 2022).
11. Katzmarzyk, PT, Friedenreich, C, Shiroma, EJ, and Lee, IM. Physical Inactivity and Non-communicable Disease burden in Low-Income, Middle-Income and High-Income Countries. Br J Sports Med (2021) 56:101. doi:10.1136/bjsports-2020-103640
12. Strain, T, Brage, S, Sharp, SJ, Richards, J, Tainio, M, Ding, D, et al. Use of the Prevented Fraction for the Population to Determine Deaths Averted by Existing Prevalence of Physical Activity: a Descriptive Study. Lancet Glob Health (2020) 8(7):e920. doi:10.1016/S2214-109X(20)30211-4
13. Hamer, M, and Chida, Y. Walking and Primary Prevention: A Meta-Analysis of Prospective Cohort Studies. Br J Sports Med (2008) 42(4):238–43. doi:10.1136/bjsm.2007.039974
14. Kelly, P, Kahlmeier, S, Götschi, T, Orsini, N, Richards, J, Roberts, N, et al. Systematic Review and Meta-Analysis of Reduction in All-Cause Mortality from Walking and Cycling and Shape of Dose Response Relationship. Int J Behav Nutr Phys Act (2014) 11:132. doi:10.1186/s12966-014-0132-x
15. Woodcock, J, Edwards, P, Tonne, C, Armstrong, BG, Ashiru, O, Banister, D, et al. Public Health Benefits of Strategies to Reduce Greenhouse-Gas Emissions: Urban Land Transport. Lancet (2009) 374(9705):1930–43. doi:10.1016/s0140-6736(09)61714-1
16. Egiguren, J, Nieuwenhuijsen, MJ, and Rojas-Rueda, D. Premature Mortality of 2050 High Bike Use Scenarios in 17 Countries. Environ Health Perspect Déc (2021) 129(12):127002. doi:10.1289/ehp9073
17. Mindell, J, Ison, E, and Joffe, M. A Glossary for Health Impact Assessment. J Epidemiol Community Health (2003) 57(9):647–51. doi:10.1136/jech.57.9.647
18. Hess, JJ, Ranadive, N, Boyer, C, Aleksandrowicz, L, Anenberg, SC, Aunan, K, et al. Guidelines for Modeling and Reporting Health Effects of Climate Change Mitigation Actions. Environ Health Perspect (2020) 128(11):115001. doi:10.1289/ehp6745
19. Toulouse, E, Le Dû, M, Gorge, H, and Semal, L. Stimulating Energy Sufficiency: Barriers and Opportunities. In: ECEEE Summer Study. Toulon: ECEEE (2017). p. 59–70.
20.Association négaWatt. Scénario négaWatt 2022 - Le scénario en détails. Alixan, France (2022). Available at: https://www.negawatt.org/IMG/pdf/scenario-negawatt-2022-rapport-complet-partie4.pdf (Accessed May 18, 2022).
21. Papon, F, and De Solère, R. Les modes actifs: marche et vélo de retour en ville. La revue, Commissariat général au développement durable-Service de l’observation et des statistiques (2010).
22.Center for Transport Analytics, Transport DTU. The Danish National Travel Survey (TU) - Latest Dataset (2022). Available at: https://www.cta.man.dtu.dk/english/national-travel-survey/latest_dataset (Accessed January 14, 2022).
23. Castro, A, Gaupp-Berghausen, M, Dons, E, Standaert, A, Laeremans, M, Clark, A, et al. Physical Activity of Electric Bicycle Users Compared to Conventional Bicycle Users and Non-cyclists: Insights Based on Health and Transport Data from an Online Survey in Seven European Cities. Transportation Res Interdiscip Perspect (2019) 1:100017. doi:10.1016/j.trip.2019.100017
24.National Institute of Statistics and Economic Studies (INSEE). Projections de population 2007-2060 pour la France métropolitaine. Insee Résultats (2010). p. 117. Available at: https://www.insee.fr/fr/statistiques/1281151?sommaire=2020101 (Accessed June 23, 2022).
25. Götschi, T, Kahlmeier, S, Castro, A, Brand, C, Cavill, N, Kelly, P, et al. Integrated Impact Assessment of Active Travel: Expanding the Scope of the Health Economic Assessment Tool (HEAT) for Walking and Cycling. Int J Environ Res Public Health (2020) 17(20):7361. doi:10.3390/ijerph17207361
26.World Health Organization. Global Recommendations on Physical Activity for Health. Geneva, Switzerland (2010). Available at: https://www.who.int/publications/i/item/9789241599979 (Accessed June 23, 2022).
27. Quinet, E. Cost Benefit Assessment of Public Investments. Final Report Summary and recommendations. Paris, France: CGSP-Policy Planning Commission (2013). Available at: https://www.strategie.gouv.fr/sites/strategie.gouv.fr/files/archives/CGSP_Evaluation_socioeconomique_17092013.pdf (Accessed June 23, 2022).
28. Bouscasse, H, Gabet, S, Kerneis, G, Provent, A, Rieux, C, Ben Salem, N, et al. Designing Local Air Pollution Policies Focusing on Mobility and Heating to Avoid a Targeted Number of Pollution-Related Deaths: Forward and Backward Approaches Combining Air Pollution Modeling, Health Impact Assessment and Cost-Benefit Analysis. Environ Int (2021) 159:107030. doi:10.1016/j.envint.2021.107030
29. Zhao, Y, Hu, F, Feng, Y, Yang, X, Li, Y, Guo, C, et al. Association of Cycling with Risk of All-Cause and Cardiovascular Disease Mortality: A Systematic Review and Dose-Response Meta-Analysis of Prospective Cohort Studies. Sports Med (2021) 51(7):1439–48. doi:10.1007/s40279-021-01452-7
30. Rissel, C, Curac, N, Greenaway, M, and Bauman, A. Physical Activity Associated with Public Transport Use-A Review and Modelling of Potential Benefits. Int J Environ Res Public Health (2012) 9(7):2454–78. doi:10.3390/ijerph9072454
31. Goel, R, Goodman, A, Aldred, R, Nakamura, R, Tatah, L, Garcia, LMT, et al. Cycling Behaviour in 17 Countries across 6 Continents: Levels of Cycling, Who Cycles, for what Purpose, and How Far? Transport Rev (2021) 42:1–24. doi:10.1080/01441647.2021.1915898
32. Fishman, E, Schepers, P, and Kamphuis, CBM. Dutch Cycling: Quantifying the Health and Related Economic Benefits. Am J Public Health (2015) 105(8):e13–e15. doi:10.2105/ajph.2015.302724
33. de Haas, M, Kroesen, M, Chorus, C, Hoogendoorn-Lanser, S, and Hoogendoorn, S. E-bike User Groups and Substitution Effects: Evidence from Longitudinal Travel Data in the Netherlands. Transportation (2022) 49:815–40. doi:10.1007/s11116-021-10195-3
34. Van Cauwenberg, J, Schepers, P, Deforche, B, and de Geus, B. Effects of E-Biking on Older Adults' Biking and Walking Frequencies, Health, Functionality and Life Space Area: A Prospective Observational Study. Transport Res A Pol Pract (2022) 156:227–36. doi:10.1016/j.tra.2021.12.006
35. Giallouros, G, Kouis, P, Papatheodorou, SI, Woodcock, J, and Tainio, M. The Long-Term Impact of Restricting Cycling and Walking during High Air Pollution Days on All-Cause Mortality: Health Impact Assessment Study. Environ Int (2020) 140:105679. doi:10.1016/j.envint.2020.105679
36. de Souto Barreto, P, Rolland, Y, Vellas, B, and Maltais, M. Association of Long-Term Exercise Training with Risk of Falls, Fractures, Hospitalizations, and Mortality in Older Adults: A Systematic Review and Meta-Analysis. JAMA Intern Med (2019) 179(3):394–405. doi:10.1001/jamainternmed.2018.5406
37. Praznoczy, C. Les bénéfices et les risques de la pratique du vélo-Évaluation en Ile-de-France. Pollut Atmosphérique (2012) 4:57.
38. Schwarz, E, Leroutier, M, Quirion, P, and Jean, K. Cycling for Climate and Public Health: A Missed Opportunity for France [Preprint]. medRxiv (2022). doi:10.1101/2022.04.14.22273838
39.Observatoire national interministériel de la sécurité routière (ONISR). Bilan 2020 de la sécurité routière (2021). Available at: https://www.onisr.securite-routiere.gouv.fr/etat-de-l-insecurite-routiere/bilans-annuels-de-la-securite-routiere/bilan-2020-de-la-securite-routiere (Accessed January 14, 2022).
40. Bonaldi, C, and Hill, C. Alcohol-attributable Mortality in France in 2015. In: Bull Épidémiologique Hebdomadaire (2019). Saint-Maurice, France: Santé Publique France p. 97–108.
41.Vélo and Territoires. Bulletins fréquentations vélo en France. Plateforme nationale des fréquentations (2022). Available at: https://www.velo-territoires.org/observatoires/plateforme-nationale-de-frequentation/bulletins-frequentations-velo/ (Accessed January 14, 2022).
42. Bernard, P, Chevance, G, Kingsbury, C, Baillot, A, Romain, A-J, Molinier, V, et al. Climate Change, Physical Activity and Sport: A Systematic Review. Sports Med (2021) 51(5):1041–59. doi:10.1007/s40279-021-01439-4
43.ADEME-Agence de la transition écologique. Transition(s) 2050. 4 Scenarios to Achieve Carbon Neutrality (2022). Available at: https://transitions2050.ademe.fr/en.2022 (Accessed January 14, 2022).
Keywords: physical activity, health impact assessment, active transportation, transition scenario, climate change mitigation
Citation: Barban P, De Nazelle A, Chatelin S, Quirion P and Jean K (2022) Assessing the Health Benefits of Physical Activity Due to Active Commuting in a French Energy Transition Scenario. Int J Public Health 67:1605012. doi: 10.3389/ijph.2022.1605012
Received: 15 April 2022; Accepted: 02 June 2022;
Published: 12 July 2022.
Edited by:
Olaf Von Dem Knesebeck, University Medical Center Hamburg-Eppendorf, GermanyReviewed by:
Nico Vonneilich, University Medical Center Hamburg-Eppendorf, GermanyKarim Abu-omar, University of Erlangen Nuremberg, Germany
Copyright © 2022 Barban, De Nazelle, Chatelin, Quirion and Jean. This is an open-access article distributed under the terms of the Creative Commons Attribution License (CC BY). The use, distribution or reproduction in other forums is permitted, provided the original author(s) and the copyright owner(s) are credited and that the original publication in this journal is cited, in accordance with accepted academic practice. No use, distribution or reproduction is permitted which does not comply with these terms.
*Correspondence: Kévin Jean, kevin.jean@lecnam.net